Semiconductor Physics: Part 6 - Manufacture
Last time, we spent some time thinking about direct and indirect bandgap materials, and considered the differences in their material characteristics. We then considered Vegard's law, which formalises the linear interpolation between two binary materials , before discussing Anderson's rule, which is helpful in determining the relative alignment of the bandgaps when two materials are grown at a heterojunction. In this post we will tackle strain.
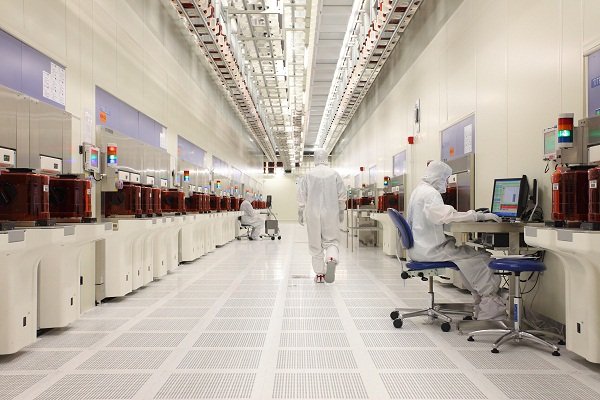
Strain
If you were to start growing a material that has a naturally larger lattice constant on top of a material that forms a smaller lattice constant, it is possible to force the lattice constants to be equal, by quite literally squeezing the bonds. At the critical distance, the strain energy is equal to the bond energy, and beyond this the lattice becomes dislocated and fractured.
Another possibility is that the lattice just evolves along its preferred oritentation, but this comes with defects, or missing bonds. If you were to grow a heterostructure with materials of different lattice constants, you either need to be a very skilled grower, and find ways to grow strained lattices such that they don't fracture, or you just accept that there will be defects at the heterojunction interface. Often these defects are bad news for electric materials, as they act as scattering centres. When growing heterostructures, mismatched epitaxy is usually not good and is best avoided where possible.
Now, there are two types of strain; namely, compressive and tensile strain, which both cause bowing. From this we get the critical thickness: you can only grown a certain amount of material if you have grown a strained lattice. Beyond the critical thickness, you get destructive wafer bowing.
It is very interesting to consider GaAs and AlGaAs; both have the same lattice constant, meaning we can add Al into GaAs almost indefinitely, and you can quite easily grow AlGaAs on top of GaAs, since the strain is matched. This opens up the door to a great wealth of possibilities for the engineering of heterostructures, quantum wells and barriers, with no thickness limitations imposed. Heterostructure engineering is made difficult when your materials are lattice mismatched, leaving you with no choice but to grow strained or defect-heavy structures.
If you take a substrate of one lattice constant, and grow a layer with a different lattice parameter, the strain builds up. You can compensate, however, if you implement a material with a smaller lattice constant in the following layer, balancing the net strain. This techniques makes possible the growth of multiple strained layers with no defects. Bragg mirrors are a common example of materials grown via this process. The only requirement for strain compensation is that each layer must be grown beneath the critical thickness, but the number of possible layers in such structures is not constrained.
Island formation can be forced if you grow materials with very different lattice constants, as atoms tend to stick to eachother better than they do the lattice, leading to the material on top buckling into small islands. These so called island defects are usually pyramidal in shape. Now, one man's defect is another man's quantum dot: these pyramidal defects are in fact quantum dots! This is the best way to grow quantum dots, and was discovered by Stranski and Krastanow in 1938, after which the growth process is named. The major drawback, however, is that island formation is a completely random process.
In the next post, we will continue along this line of thought and begin to consider self organised quantum dot systems and quantum wire growth.