Semiconductor Physics: Part 2 - Nanostructures
This post will follow on from our previous discussion on length scales. We saw previously that the nanometer length scale matched up nicely with the de Broglie wavelength of semiconducting electrons and holes. This means we can observe quantum effects on this length scale.
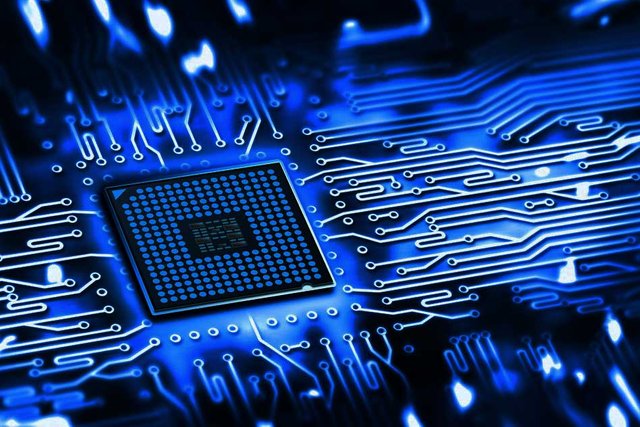
The Bohr Radius
The closest stable 'orbit' of an electron around the nucleus in a planetary fashion is the Bohr radius,
where e0 is the permittivity of free space, hbar is the reduced Planck constant (h/2pi), q is the charge of the electron and m0 is the electron rest mass. The Bohr radius, a0 is of the order 0.05 nm.
We can deduce the Bohr radius by equating the centripetal force of the electron to the Coulomb interaction,
Now, for an eigenstate,
Equating this with the de Broglie wavelength, and noting that p = mv, we have
which leads us to
So we see, that the Bohr radius is crudely the radius at which the electron orbits the stationary nucleus, for n = 1 ground state.
The Exciton
The simple model of an exciton is a two particle captured electron - hole pair; one whizzing around the other, kept there by Coulomb attraction.
There are two types of exciton, distinguished by their binding energies - Frenkel excitons (~100 meV) and Mott-Wannier excitons (~10 meV).
The electron and hole orbits in a dielectric medium (essentially a centre of mass system), with the total Bohr radius of the exciton
We introduce the reduced mass
Allowing us to write the exciton Bohr radius as
We note that the total energy of the exciton must be the sum of its kinetic and potential energies, confine motion to the x direction, and apply equation (2) with a modified dielectric constant to yield
This is the total energy of the exciton.
Confinement Regimes
The Coulomb attraction is dependent upon 1/r, and the nanoscale confinement energy is dependent upon 1/r^2. The latter grows very quickly when the size of the system becomes small.
Weak Confinement
This is the case where the dimension of the nanostructure, a > a (electron), a (hole). In this case, the exciton binding energy is weak (as in bulk systems) and the optical and electrical properties are ~ bulk like.
Strong Confinement
This is the case where the dimension of the nanostructure, a < e (electron), a (hole). In this case, the exciton binding energy is large, and optical and electrical properties are dominated by confinement.
Intermediate Confinement
This is the case where the dimension of the nanostructure, a < e (electron), but > a (hole). It is in this case where quantisation effects start to become observable.
De Broglie Wavelength
Consider the Schrodinger equation,
If we constrian the wavefunction to reside within an infinite potential well of length L, for which psi(0) = psi(L) = 0, and let
Then we may rewrite equation (10) as
which has general solution
From the boundary conditions, we have B = 0 and k = n pi/L, therefore
Now, the Heiseberg uncertainty principle tells us that the uncertainty in the position and momentum are related via
Therefore in order to see quantum effects, the thermal energy (kT/2) must be comparable to the energy of confinement, and we must have
In the next post, we will discuss the manufacture of low dimension semiconductor devices.