Biology The Study of Life: Part 20 (Darwin VS Mendel)
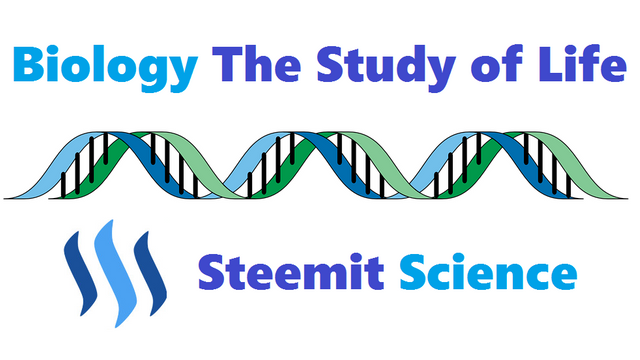
Introduction
This informative series of posts will explore modern biology; the fundamental principles of how living systems work. This material will always be presented at the level of a first-year college biology course, without assuming any prior background in biology or science. It also presents material in a conceptual format. Emphasizing the importance of broad, unifying principles, facts and details in the context of developing an overarching framework. Finally, the series takes a historical approach wherever possible. Explaining how key experiments and observations led to our current state of knowledge and introducing many of the people responsible for creating the modern science of biology.
This post begins by discussing the apparent conflict between Mendel’s work and Darwin’s theory of natural selection.
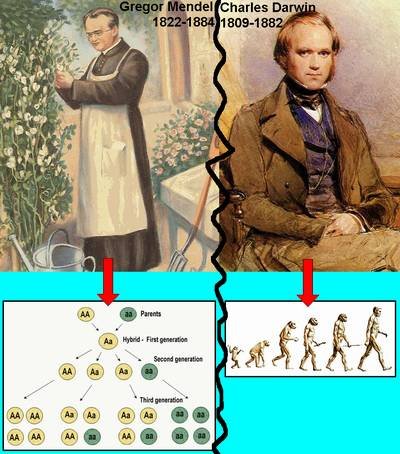
The resolution to this conflict was achieved eventually through the modern synthesis, which depended on formal methods for modeling gene frequency changes in populations. The post goes on to illustrate how the gene pool of a population can be determined for a simple trait. This exercise leads to a discussion of how evolution, formally measured as a change in the relative frequency of genes across generations, can be modeled with a few simple equations. The post continues by describing the Hardy-Weinberg equilibrium, which predicts that evolution will not occur in a sexually reproducing population. The resolution to this apparently paradoxical conclusion comes from understanding that assumptions underlying the Hardy-Weinberg equilibrium are typically violated in natural populations.
After Darwin’s Origin of Species was published in 1859, the scientific community generally accepted the fact of evolution.
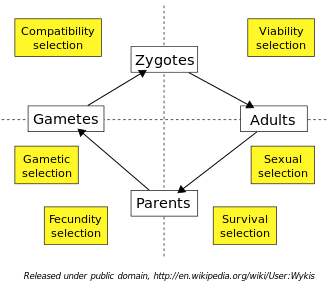
The mechanism that Darwin proposed, natural selection, was also accepted at first but lost favor when Mendelian genetics reemerged in the early 20th century. One reason Darwin’s ideas about natural selection lost favor was that Darwin had not suggested a convincing mechanism by which heritable traits might be transmitted from parents to offspring. Mendelian genetics did provide a mechanism for trait transmission, one that corresponded to the movement of chromosomes, which were just beginning to be identified as physical carriers of genetic information. Mendelian genetics seemed to be at odds with Darwin’s thinking, however. Darwin emphasized gradual change in traits that showed continuous variation in a population. By contrast, Mendel and his followers successfully modeled the transmission of discrete, dichotomous traits. The Mendelians argued that natural selection for very small differences among traits could not account for the patterns of trait transmission they studied.
The eventual resolution of these two perspectives, which is referred to as the modern evolutionary synthesis, came much later, in the 1930s and 1940s.
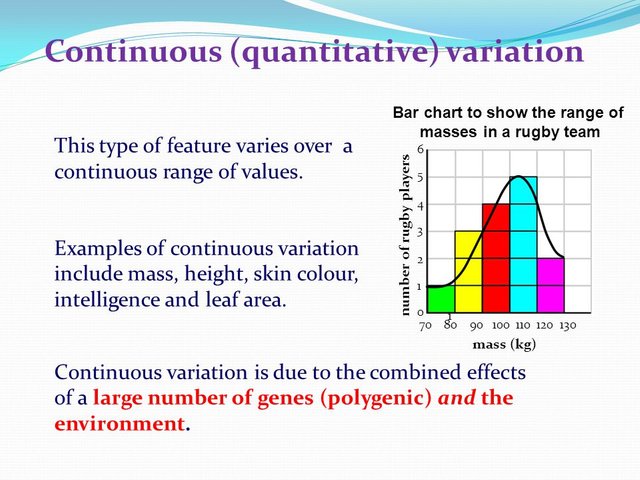
One key to the modern synthesis was the realization that not all traits followed Mendelian rules of inheritance for single genes. Most notably, traits exhibiting continuous variation, such as height or weight, depend on potentially, many genes, all acting in concert.
Another key to the modern synthesis was the development of formal methods (in a field called population genetics) to model gene frequency change in populations.
The relationship between genotype and phenotype is normally not as straightforward as the discussion of Mendelian genetics might make it seem.
The first set of complications arises from the fact that alleles can interact in more complex ways than in Mendel’s pea plants. Snapdragon flowers that are homozygous for red and white colors, for example, will have an F1 generation with all pink flowers, because the heterozygous genotype for color has its own unique phenotype. This kind of interaction is called incomplete dominance. Many traits have more than two alleles, such as in the human ABO blood-type system. Types A and B are both dominant over O, but codominant to each other. Codominance means that both alleles are expressed equally.
Genes can also interact with each other, as in epistasis, where the expression of one gene depends on an entirely different gene. For example, mice have alleles for brown or black fur, but an entirely different gene controls whether any pigment is deposited into the fur in the first place.
The third and most ubiquitous set of complications is the result of the environment on gene expression. There are few if any cases where the environment does not play a role in gene expression.
The final cause of the complexity of this relationship is that for many heritable traits, phenotypical expression depends on the cumulative effect of many different genes.
Instead of being discrete, dichotomous traits, they are continuously variable and must be measured (quantified) on some scale hence their name, quantitative traits.
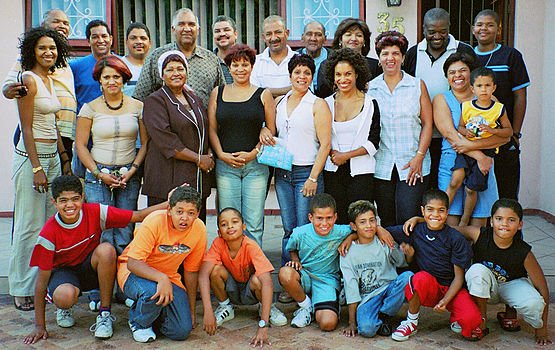
Skin color in humans is a quantitative trait. There is evidence that skin pigmentation is affected by at least three genes, each with two alleles, one for darker skin and one for lighter skin. A person with six dark alleles will have the darkest skin; a person with zero dark alleles will have the lightest skin, and a person with three dark alleles will be somewhere in between. Although this is a simplification (there are obviously more than seven gradations of skin color), it shows the additive effect of multiple genes. The realization that the cumulative action of multiple genes, each following Mendelian principles, could produce continuously variable expression paved the way for the synthesis of Mendelian genetics with Darwinian natural selection.
How can we begin to expand genetic models to account for the evolution of traits in populations?
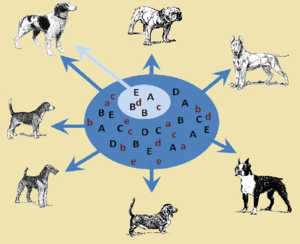
In this context, a population is a group of individuals of the same species, all of which may mate with each other. The aggregate genetic makeup of this population is called its gene pool, which consists of all the alleles for all the genes for all the individuals in the group. In Mendelian genetics, the possible genotypes of offspring depended on the alleles present in the parents.
In population genetics, the genotypes of future generations depend on the gene pool as a whole and on the relative frequencies of all alleles in the pool. In population genetics, evolution is a change in the relative frequencies of alleles in a gene pool for a particular population.
As an example, the ability to roll the human tongue is a trait controlled by a single gene;
it illustrates how we can determine allele frequencies in a gene pool based on our knowledge of phenotypes and the relationship between phenotypes and genotypes.
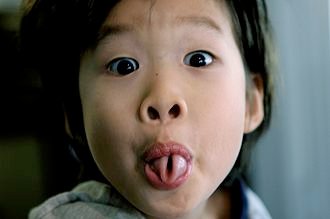
- In a typical human population, about 91% of individuals can roll their tongues, and about 9% cannot. These percentages are the frequencies of phenotypes in the population.
- Each human has two alleles for this gene; thus, for a population of 100 individuals there are 200 alleles in our gene pool.
- It is hard to determine the relative frequencies of alleles directly, because “rollers” can have either the TT or Tt genotype, given that the tongue-rolling allele (T) is dominant over the non-rolling allele (t).
- “Non-rollers,” though, can have only the tt genotype; using this information, we can calculate the relative frequencies of all other alleles: 70% of the total alleles would be T alleles and 30% would be t alleles.
- The Hardy-Weinberg equation uses simple probability theory to compute the relative proportions of T and t alleles in the offspring of this population. It says that mating essentially draws alleles from the gene pool at random.
- The probability of an offspring having a particular two-allele genotype is calculated by multiplying together the probabilities of picking either allele. For example, the probability of a TT genotype is 0.7 x 0.7 = 0.49, or 49%.
- The Hardy-Weinberg equation predicts that 49% of our population will have the TT genotype, 42% will have the Tt genotype, and 9% will have the tt genotype. Because T is dominant, the TT and Tt genotypes can both roll their tongues. We can see that the equation works (49% + 42% = 91%).
This equation allows us to predict allele frequencies in successive generations of offspring, but we will reach a point where the frequencies will not change from generation to generation.
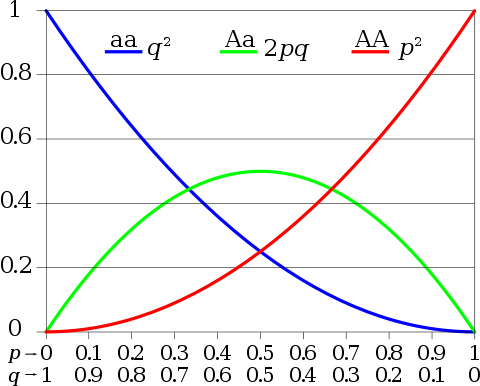
This point is called the Hardy-Weinberg equilibrium. This equilibrium seems puzzling at first, because it suggests that no evolutionary change can occur. Genetic recombination only shuffles existing alleles and, thus, has no effect on the relative frequencies found in a gene pool. The answer to this paradox is that the Hardy-Weinberg equation, like any model, rests on a number of specific assumptions. Identifying these assumptions and understanding when and how they are violated in natural populations provides a framework for predicting when and how evolution will occur.
END PART 20
BIOLOGY THE STUDY OF LIFE:
PART 1 INTRODUCTION
PART 2 WHAT IS LIFE
PART 3 ORIGIN OF LIFE
PART 4 CELL TO ORGANISM
PART 5 PROTEINS
PART 6 CODE OF LIFE
PART 7 DOUBLE HELIX
PART 8 REPLICATING DNA
PART 9 CENTRAL DOGMA
PART 10 GENETIC CODE
PART 11 DNA TO RNA
PART 12 RNA TO PROTEINS
PART 13 MISTAKES HAPPEN
PART 14 WHEN CELLS DIVIDE
PART 15 MENDEL'S PEA PLANTS
PART 16 SEX AND VARIATION
PART 17 GENES AND CHROMOSOMES
PART 18 CHARLES DARWIN ON THE ORIGIN OF SPECIES
PART 19 NATURAL SELECTION
PART 20 DARWIN VS MENDEL
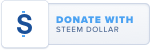



Image Credits:
ALL IMAGES UNLESS NOTED - Wikipedia