Biology The Study of Life: Part 7 (Double Helix)
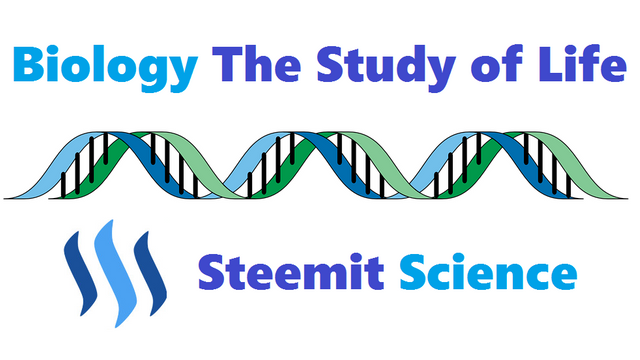
Introduction
This informative series of posts will explore modern biology; the fundamental principles of how living systems work. This material will always be presented at the level of a first-year college biology course, without assuming any prior background in biology or science. It also presents material in a conceptual format. Emphasizing the importance of broad, unifying principles, facts and details in the context of developing an overarching framework. Finally, the series takes a historical approach wherever possible. Explaining how key experiments and observations led to our current state of knowledge and introducing many of the people responsible for creating the modern science of biology.
This post continues the story of DNA from its identification as the information-carrying molecule in cells to the discovery of its three-dimensional structure.
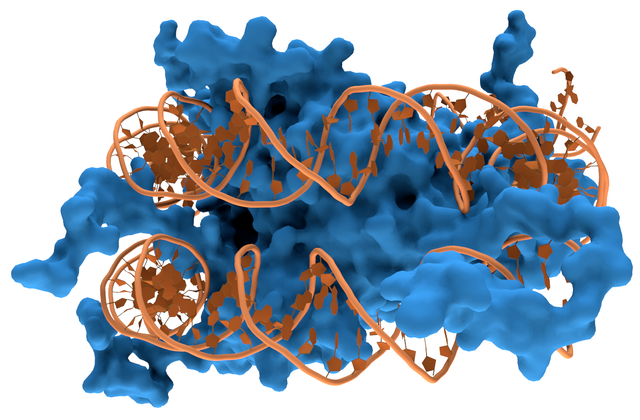
Beginning with a discussion of why researchers focused on the structure of DNA in the first place, then outlining how the experimental evidence of Franklin, Wilkins, and others led to discovery of the double helix by Watson and Crick. The post ends by discussing the most important significance of the discovery of the double helix, which was to suggest a mechanism by which the information in DNA can be accurately replicated, solving one of the greatest problems in modern biology.
Hershey and Chase’s conclusion that DNA is the genetic material led to one of the most famous races to discovery in the history of biology.
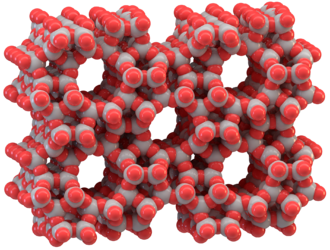
Because both proteins and DNA are polymers with variable groups, it seemed intuitive that there was a mapping between the two. However, DNA’s linear structure did not reveal any clues about how DNA could be replicated. Successful work on the relationship between protein structure and function led scientists to believe that understanding the three-dimensional structure of DNA might reveal its replication mechanism.
In x-ray crystallography, diffraction patterns produced by x-rays passing through a crystallized substance provide clues about the physical structure of the substance. Rosalind Franklin and Maurice Wilkins, working at Kings College in London, were able produce well-resolved x -ray crystallographs of very pure crystallized DNA. They were not, however, able to fully deduce the three-dimensional structure of DNA from the clues provided by their own crystallographs.
Another source of insight came from the work of Erwin Chargaff, who performed biochemical analyses of the relative amounts of different nitrogenous bases in samples of DNA from many species. Chargaff discovered that, in any sample of DNA, the amount of adenine (A) always equals the amount of thymine (T), and the amount of cytosine (C) always equals the amount of guanine (G). These equivalencies of the amounts of A and T and the amounts of C and G, known as Chargaff’s Rule, were considered curious, and the significance of this finding was not immediately apparent.
James Watson and Francis Crick, working at Cambridge University, deduced the three-dimensional structure of DNA from insights they obtained from Franklin and Wilkins’s x-ray crystallograph of DNA and from Chargaff’s Rule.
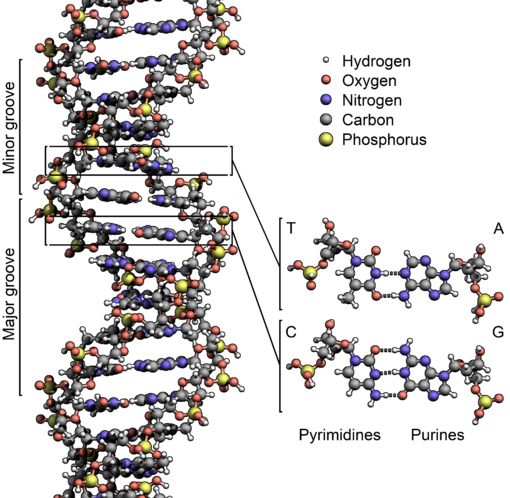
Watson realized the significance of Franklin and Wilkins’s data when he saw their findings on a lecture trip to London; he noted some key dimensions from the crystallograph, and he and Crick began formulating several physical models that might conform to these data. The x-ray data made it clear that DNA normally occurs as a double-stranded helix and that each turn of the helix contained 10 nucleotides. Watson and Crick made physical models of DNA to test whether different helix formations conformed to their observations. Watson and Crick realized that the nitrogenous bases must go on the inside of the helix, which makes sense given that the bases are hydrophobic and would tend to face inward. They also realized that the two strands of a DNA molecule are antiparallel (that is, they run in opposite directions).
“Complementary base pairing” provided the last piece of the puzzle. Because purines and pyrimidines are different sizes, random base pairs across the helix would give DNA a variable width, which is unstable. Chargaff’s Rule helped Watson and Crick realize that purines must always pair with pyrimidines and, further, that A must always pair with T, and G must always pair with C.
In 1953, Watson and Crick published a short paper describing their model for the three-dimensional structure of DNA.
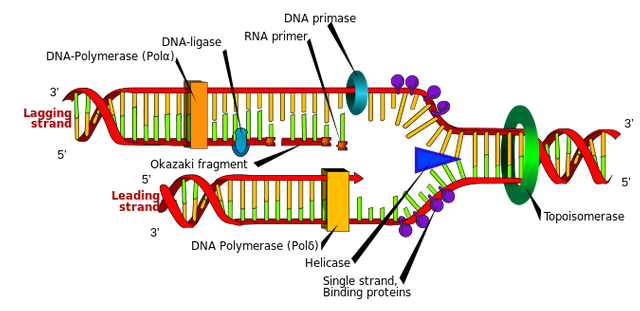
More significant than the structure of DNA itself was the fact that, as expected, this structure suggested a mechanism whereby DNA could be accurately replicated. Watson and Crick’s paper ends with “It has not escaped our attention that the specific pairing we have postulated immediately suggests a possible copying mechanism for the genetic material.” The complementary base pairing of A with T and G with C suggested that the double helix could unwind into two single strands of DNA, each of which could serve as an unambiguous template for the complementary strand.
Watson, Crick, and Wilkins, but not Rosalind Franklin, won the Nobel Prize for Physiology and Medicine in 1962 for the discovery of DNA’s double helix.
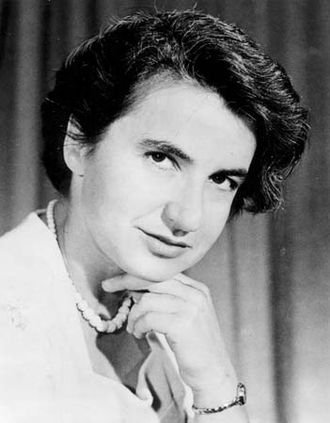
Some have suggested that Franklin was denied credit for what may be the single most important contribution to the discovery of the double helix. Franklin had a difficult relationship with Maurice Wilkins and her colleagues at Wilkins’s lab; she was asked to leave and cease work on DNA altogether. She eventually did so and began to work on viruses. Accounts of the discovery of DNA from the 1960s and 1970s minimize Franklin’s contribution and emphasize Watson and Crick’s work. The main reason Franklin did not win a Nobel Prize, however, is that she died in 1958, and Nobel Prizes are not awarded posthumously. Fortunately, her contributions are now more fully recognized and taught.
Watson and Crick suggested that the DNA double helix must “unzip,” and new complementary strands must form from the originals.
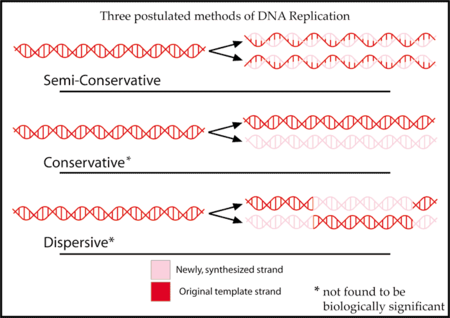
Two alternatives to this model were proposed.
1. The conservative model suggested that the double helix remained intact and was used whole as a template for copies.
2. The dispersive model suggested that the original DNA molecule was completely broken down during replication and the new copy was created by some unknown mechanism.
Based on what was known in the 1950s, both of these alternatives were completely likely, though they seem improbable now. They still retain value, though, as ways to compare predictions made by Watson and Crick’s model. Watson and Crick’s model became known as the semiconservative model, because the DNA is disassembled during replication, but the original strands are incorporated whole in the new copies.
The competing models each predicted a different mixture of genetic material after a copying process.
1. The semiconservative model leads to a prediction that each new DNA molecule would be made of one original strand and one freshly created strand.
2. The conservative model predicts that new copies would contain freshly created strands but that the originals would also remain intact.
3. The dispersive model predicts that the original DNA would be destroyed and its components randomly scattered across the strands of the new copies.
Although distinguishing original DNA from new copies is conceptually straightforward, it took several years for anyone to figure out how to accomplish this experimentally.
END PART 7
BIOLOGY THE STUDY OF LIFE:
PART 1 INTRODUCTION
PART 2 WHAT IS LIFE
PART 3 ORIGIN OF LIFE
PART 4 CELL TO ORGANISM
PART 5 PROTEINS
PART 6 CODE OF LIFE
PART 7 DOUBLE HELIX
PART 8 REPLICATING DNA
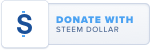
If you enjoy high quality educational content, please consider donating.



Image Credits:
ALL IMAGES UNLESS NOTED - Wikipedia
This is so exciting to come across your article and can't wait to read the next! thank you for your dedicated good work and sharing. Namaste :)
Science is oftentimes an "old mans club" and Rosalind Franklin is just one of many glaring examples of a brilliant woman not getting the recognition she deserves for her contributions to the advancement of scientific knowledge ( I think she deserved to be apart of that Nobel Prize!).
Yes, I agree she deserved to be awarded the Nobel Prize and even if posthumous awards are not given by the Nobel Committee an exception should have been made in this case.
It causes me a real pleasure to read your post excellent congratulations
It is a shame that Franklin did not get the recognition she deserved, but I assume there are a lot of examples of unrecognised achievements in the history of science.
If you are interested in history, see my Today in History article.