Biology The Study of Life: Part 11 (DNA to RNA)
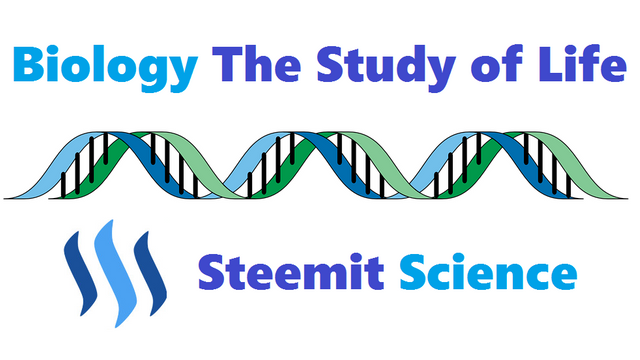
Introduction
This informative series of posts will explore modern biology; the fundamental principles of how living systems work. This material will always be presented at the level of a first-year college biology course, without assuming any prior background in biology or science. It also presents material in a conceptual format. Emphasizing the importance of broad, unifying principles, facts and details in the context of developing an overarching framework. Finally, the series takes a historical approach wherever possible. Explaining how key experiments and observations led to our current state of knowledge and introducing many of the people responsible for creating the modern science of biology.
This is the first of two posts that complete the description of the journey of genetic information from DNA to functional proteins.
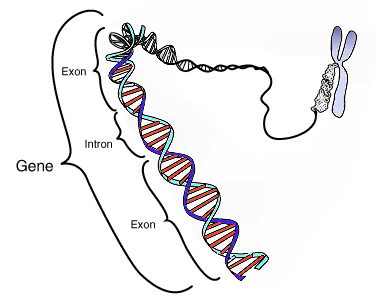
It begins by describing the key features of how DNA is transcribed into RNA. It then goes on to show how initial transcriptions of RNA are cut up and pasted back together to produce a functional message. Introns are stretches of DNA that lack obvious coding function. Introns are excised from the initial transcript, and the remaining exons are stitched together to produce a contiguous message, leaving much of the original transcript on the cutting-room floor. Armed with this background, we conclude the post by revisiting the molecular definition of a gene.
The molecular mechanisms of transcription (the synthesis of RNA) are conceptually quite similar to those involved in replication (the synthesis of new DNA), largely because RNA and DNA are very similar molecules.
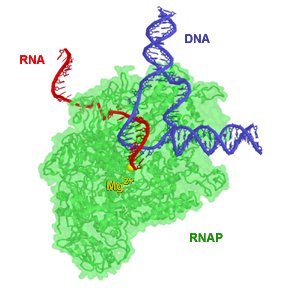
RNA is used as an intermediate, or messenger, passing information from DNA to the protein-synthesis process. The key enzyme involved in transcription is RNA polymerase (RNAP), which acts much like DNA polymerase. As in replication, the DNA double helix must first unwind, after which RNA polymerase uses complementary base pairing to synthesize a polymer that maintains information as a unique sequence of nucleotide bases.
There are some important differences between replication and transcription.

RNA polymerase requires fewer enzymes to work than DNA polymerase. For example, RNA polymerase typically opens the double helix itself without the help of helicase. Only one of the two DNA strands is copied during transcription because only one sequence of nucleotides is needed to code for amino acids. The transcribed strand is called the template, or coding strand; the opposite strand is called the complementary strand. The template strand, however, is not always on the same side of the double helix, and the distribution of meaningful information on an articular side of the helix is random. A complementary strand is unlikely to be a template strand for another gene because the constraints of complementary base pairing make such compactness difficult.
In transcription, only selected pieces of DNA are transcribed, not the entire molecule.
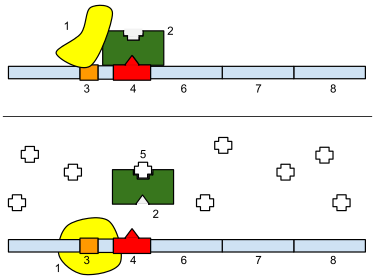
Specific nucleotide sequences, called promoters, mark the beginning of transcription for each gene, and other sequences, called termination sites, signal the end of each gene. (Start and stop codons are instructions to the protein synthesizers, not RNA polymerase.) Together, a promoter, termination site, and the DNA in between are called a transcription unit.
During replication, only one copy of the DNA molecule is made. During transcription, a single gene may be copied hundreds or thousands of times, depending on the needs of the protein-synthesis process. RNA polymerase does not on its own recognize promoters; instead, particular stretches of DNA to be transcribed are specified by other proteins that recognize them. The presence or absence of these transcription factors serves as a kind of switch to control when stretches of DNA are to be transcribed into RNA.
DNA contains quite a bit of apparently useless material whose purpose is not known.
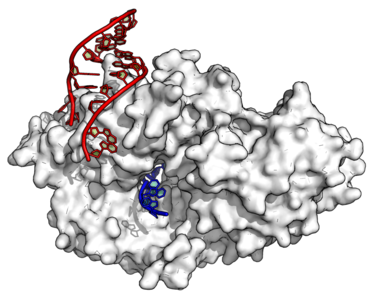
In eukaryotes, the initial RNA transcript includes large segments of RNA that are not part of the code needed to produce a protein. These stretches must be eliminated somehow in order to make a fully functional transcript for the protein. The average size of a transcription unit is about 8,000 nucleotides long. Once the noncoding nucleotides are removed, the average size of a transcript is about 1,200 nucleotides.
This means that as much as 85% of the transcribed mRNA is cut out and not translated!

The pieces of RNA that are cut out and thrown away are called introns, while the remaining pieces that are spliced together are called exons. The excision of introns and splicing of exons to form a final RNA transcript is done by a set of enzymes and catalytic RNAs known as snRNPs (small nuclear ribonucleoproteins), which act together as a spliceosome. snRNPs identify the ends of introns, break the RNA at these locations while maintaining a connection with the adjacent RNA pieces, then bring these pieces together physically and rejoin the broken strand. The end product is a mature mRNA strand that includes only exons.
In addition to having introns spliced out, the ends of eukaryotic RNA strands are also chemically modified before the strands are exported from the nucleus.

At the 5' end of the polarized RNA molecule, a modified form of guanine is added (the guanine cap); at the 3' end, a string of several hundred adenines (the polyA tail) is added. The guanine cap and polyA tail appear to have two functions. First, they stitch up the ends of the RNA molecule, protecting it from degradation by enzymes that normally break down RNA. Second, they appear to increase the efficiency with which the final RNA transcript is translated into protein after it has left the nucleus.
Several hypotheses have been advanced to explain the presence of introns.

- One possibility is that the splicing process controls the rate and/or timing of processing mRNA strands.
- Introns may also be genetic hitchhikers that serve no purpose and have been introduced into DNA in some way, possibly through retroviruses.
- In some organisms, the same transcription unit can produce different proteins depending on which parts are excised—one gene’s introns may be another gene’s exons.
- Many different genes share the same exons, which may be interchangeable structural functions (functional domains), similar to interchangeable machine parts. Introns may be a way of separating common functional domains for easy reuse.
Our discussion to this point makes it clear that, even on the molecular level, we can define a gene in several ways.
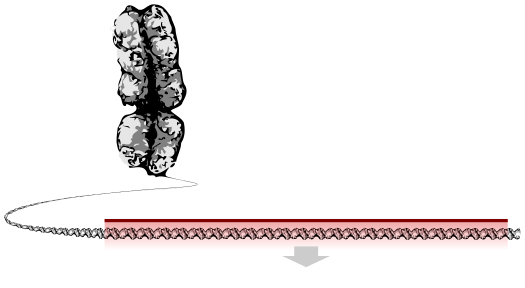
Clearly and most simply, a gene is a physical length of DNA that codes for a protein, but even this simple definition is complicated by the fact that some regions of DNA associated with genes play a regulatory role, while other regions are spliced out and thrown away.
In recent times the human genome has been completely sequenced.
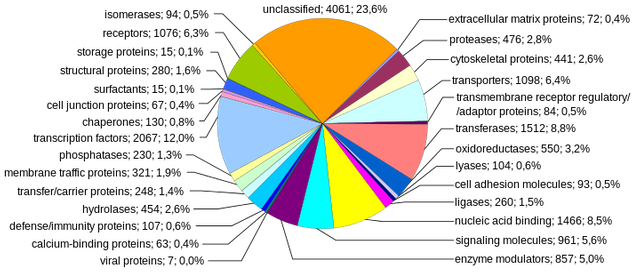
There still remains a significant challenge to determine the function of much of that sequence. This is especially a challenge for eukaryotic organisms as compared to prokaryotes.
END PART 11
BIOLOGY THE STUDY OF LIFE:
PART 1 INTRODUCTION
PART 2 WHAT IS LIFE
PART 3 ORIGIN OF LIFE
PART 4 CELL TO ORGANISM
PART 5 PROTEINS
PART 6 CODE OF LIFE
PART 7 DOUBLE HELIX
PART 8 REPLICATING DNA
PART 9 CENTRAL DOGMA
PART 10 GENETIC CODE
PART 11 DNA TO RNA
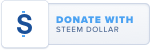



Image Credits:
ALL IMAGES UNLESS NOTED - Wikipedia