PPCC7 - basic principles for detecting elementary particles with onions
This is finally the next-to-last episode of my particle physics crash course (PPCC, you now have the meaning of the weird abbreviation that I have introduced in the title) for Steemit.
This comes just in time as I will lecture this topic to Steemians, live, next Friday at CERN, in the context of the first steemSTEM meetup where Steemit will be brought down inside the Large Hadron Collider at CERN.
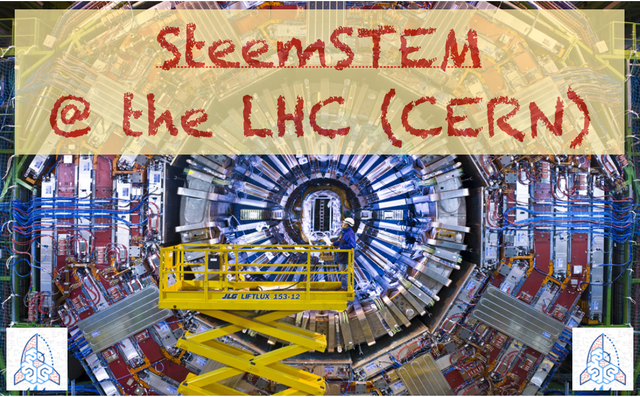
After having discussed the theoretical framework of the Standard Model of particle physics (#1, #2, #3), how to use it to make predictions (#4), the challenges attached to the hunt for new phenomena (#5) and how particle accelerators work (#6), it is now time to discuss the detection of particles through the hints that they leave in detectors.
To come back to my title, I will not discuss onions here. I leave that for the next post (that is the last one of this series). I prefer to first provide the basic principles that will help us to understand the onionish part of particle detection in the next post.
And for this, we need first to classify them into two categories: stable and visible particles, and the rest. Yes nothing fancy here… But the ways in which those particles are detected are different.
DETECTING STABLE PARTICLES - ELECTRONS, MUONS AND PHOTONS
The stable particles that I consider here consist in electrons, muons and photons, the latter two taken together with their antiparticles (i.e. positrons and antimuons). Those particles will not further decay in anything else, and they can be observed as such, provided that we have the appropriate detectors.
For the discussion here, I will treat electrons and positrons together, and generically call them electrons. Similarly, I will treat muons and antimuons together, and call them muons. The actual sign of the electric charge of the particles does not really matter.
I imagine that some may shout here (please do so, I will be pleased)… Yes, I said that muons are stable. In doing so, I have actually contradicted the first sentence of the second paragraph of the Wikipedia article on muons… and shocked hopefully most.
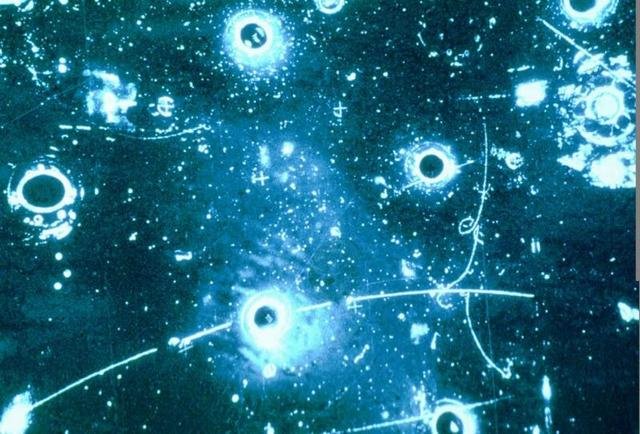
[image credits: CERN]
But I cheated a little bit. Wikipedia is not wrong here. I am however not wrong as well. I should have said that muons are somehow stable instead. The ‘somehow’ matters.
The key point is that detectors have a finite size, and muons can travel through them without decaying. They have indeed a certain lifetime that allows them to travel for several kilometers before decaying. Muons will thus decay, but far outside typical particle physics detectors.
From a detector standpoint, muons are thus stable. This was my point.
DETECTING UNSTABLE PARTICLES
There is however a lot of fundamental particles. Not only the three I mentioned in the previous section. They can be detected, but to understand how, let us classify them into three categories.
We have first unstable particles that will decay, within a typical detector, into other particles. For instance, a neutral weak Z-boson will decay about 3% of its time into an electron-positron pair. The decay products, or the daughter particles, can be detected, and by studying and measuring their properties, we can reconstruct the Z-boson and its properties.
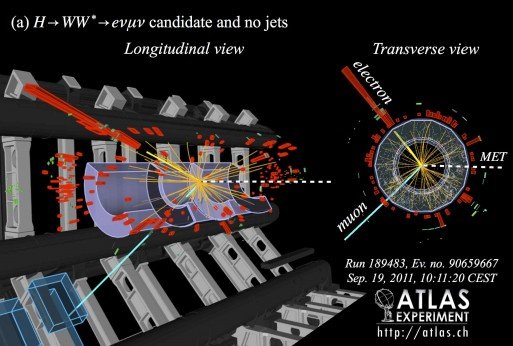
[image credits: ATLAS@CERN]
Detecting W-bosons, top quarks, tau leptons and most hypothetical particles works in the same manner. The mother particle is reconstructed from the daughter particles.
One can imagine here the difficulties related to the task of understanding what happened in a real collision. Or in other words, how to distinguish the production of a Z-boson that decayed into an electron-positron pair from the direct production of the same pair of particles? On a collision-by-collision basis, there is no way to tell…
However, statistics saves us. After accounting for a large statistics of collisions, we know exactly the signal (a real Z boson) and background (direct electron-positron production) rates and can check that the sum agrees with data. By imposing extra requirements on the final-state products, it is then possible to reduce the background (without too much impacting the signal).
STRONGLY INTERACTING PARTICLES
A second class of particles that are not detected directly consist of the strongly-interacting quarks and gluons.
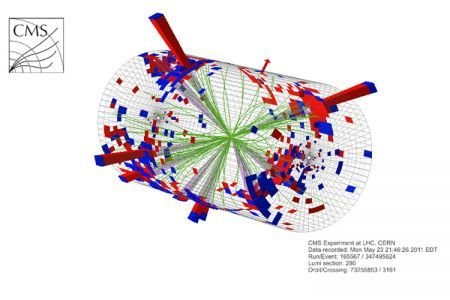
[image credits: CMS@CERN]
Those particles do not exist as such in nature, by virtue of quantum chromodynamics, the theory of the strong interactions.
Quarks and gluons indeed confine, which means that they will interact with each other and form composite states fast enough so that the only things we can observe are these bound states.
There is a full zoology of mesons (quark-antiquark pairs) and baryons (triple-quark systems) that can decay into each other, and the important point is that by doing so, they form a so-called jet of hadronic particles.
Such a jet can be detected with an appropriate detector, as illustrated in the figure on the right where 4 jets can be guessed (even if ones has no idea of what is going on on this figure, we can notice four prominent stuff…).
DETECTING THE INVISIBLE
Finally, the last class of particles that can be seen in a detector cannot actually be seen in a detector. I love this sentence…
Although my speech may sound weird, the invisible (made of neutrinos in the Standard Model) can in fact be seen. How? Well, once again, indirectly.
One golden rule in physics (and in science in general) consists in the conservation of energy. No matter what is going on, energy and momentum have to be conserved at any time.
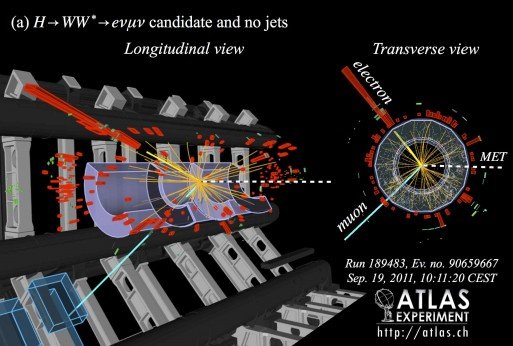
[image credits: ATLAS@CERN]
And this is the key point.
We exactly know the energy and the momentum of the colliding particles (i.e. the initial state).
Therefore, if something is missing in the detector (i.e. in the final state), this simply means that we have an invisible neutrino (or more than one) flying around and carrying a fraction of the available energy and momentum.
By measuring the properties of the visible, we can hence reconstruct the properties of the invisible. An example is in the picture on the left, where the dashed lines is the joint contribution of two neutrinos.
In the case where we would manage to produce dark matter, we will be able to reconstruct its properties in the same way, as dark matter is invisible for an LHC detector. This is one of the key point in dark matter searches at the LHC.
SUMMARY
In this post, I started to discuss how we can detect different elementary particles. Some of them can be directly detected (electrons, muons, photons) but some others cannot, either because they are unstable (and must thus be reconstructed from their decay product), or because they form jets of strongly-interacting particles (and we must thus rely on dedicated detectors) or because they are invisible (and thus reconstructed from the visible).
In the next and last episode, of this series, I will explain the role of onions in the detection of these particles. I am not kidding :D
More information can be found on the CMS website and ATLAS websites, for those interested.
SteemSTEM
SteemSTEM is a community-driven project that now runs on Steemit for more than a year. We seek to build a community of science lovers on Steemit and to promote well written/informative Science Technology Engineering and Mathematics (STEM) postings in order to make Steemit a place for fascinating STEM content.
More information can be found on the @steemstem blog and on the Steemit chat and in our last project report.
So amazing.
Very high energy colliding head on and detecting particles flying around at mind boggling speeds that are much too small to be seen, to investigate invisible ghost particles that are impossible to be seen, and also tiny unseeable particles that are never alone, very accurately calculated by a bunch of intelligent human beings made of all those weird little particles.
Our universe is strange indeed.
What are some of the equations that are most relevant for your work there?
There are many equations. For instance, if you really want to know what is going on at the hardest level (i.e. where one expects new particles to be produced), the model Lagrangian is the equation that matters (it could be the Standard Model or any model you may dream of). Strong interactions is very important, as we are colliding very energetic protons. Them if you are interested on the detector side, how visible particles interact with matter matters. And so on. There are many very relevant equations all over the place, and all are necessary at the end of the story.
@lemouth you are gradually building my interest in physics.. I hated physics because my teachers made it look so difficult and uninteresting but now I am generating an interest I wish I had a long time ago.. this is the first time I've come across the term "muons"
Muons are well known particles for about 85 years. Those can be seen as heavy electrons, to summarize that by a lot. They are produced in cosmic rays, for instance. I recommend to start checking the wikipedia page for more information, and I will then be happy to answer any further question.
Particle physics is getting out of hand now, I must say!
Piece of cake :D
After all the smashing and bashing of particles, how do you know when a new particle has been created?
There is no way to know for a given collision. However, for a statistically large number of events, we can make a selection so that we enhance the amount of signal (the particle of interest) in it. In other words, only the presence of the particle could explain data. The challenge consists in designing the selection well enough.
Really cool article @lemouth ! A couple of questions tangential to the whistle-stop tour of particle detection:
What makes the muon decay lifetime so much longer compared to the more unstable particles you describe?
Is there a process/system in place at CERN & other particle physics labs which enables some kind of "indepedence of pre-decided measurement bias"?
This is a bit hard for me to explain here, but I'll attempt it anyway:
I think I'm semi-correct in saying that In particle physics measurements, the detectors have some kind of filter in place to minimize the amount of extraneous noise being processed, so that the only set of signals being passed on for digital signal processing ("DSP") fall in within the scope of the sorts of signal sets that are being looked for (otherwise there'd be too much data to process).
....If this is the case: is there any scope for allowing for a random check of the noise data to be sampled?
Reading what I've just written makes it almost obvious that that approach isn't practical, because if the additional un-wanted "noise" is present, what could be in place to make any kind of decisions as to whether or not something novel is being found....
The issue I'm really struggling to articulate here is: is there a risk that in filtering out the "fire hose" of data before it ever reaches the DSP, serendipitous discovery becomes highly unlikely?
This has been bugging me for 35 years by the way, ever since I first read about particle physics when I was in my teens, so an answer would be very much appreciated :)
Thanks for passing by!
Special relativity. You can calculate its lifetime and the fact that at high speed, time is dilated and lengths are contracted.
A fraction of the recorded collisions are just randomly recorded. Those are the so-called minimum bias events, which allows to verify that we have not missed anything (this should also answer the second part of your comment).
I would love to attend this type of events and be able to learn what you teach. Thank you for always sharing your information with the community. Faithful follower
Maybe the next one? Unfortunately, we are full for this meetup (there is a limited number of people who can go down to the LHC).
wow very excellent
Thank you!
nice post I like @lemouth
Thanks @adeek
an interesting article discussion, this adds new insights. thanks for sharing @lemouth
It was my pleasure ^^
Super informing thank you 🙏
You are welcome!