Hawaiian volcanoes - Part 13: The Double Volcano Chain
Hawaiian volcanoes are explained as the products of a mantle plume and Hawai’i is the archetypical example of intraplate volcanism. It forms the longest island chain on Earth, with a length of nearly 6000 km and represents a time interval of more than 80 million years. The chain is generated by the movement of the Pacific plate over the Hawaiian hotspot. During the past 5 million years, the Hawaiian Islands and other volcanic islands in the Pacific have formed two parallel chains. At Hawai’i these two chains differ in their geographic location as well as their geochemistry (Tatsumotot, 1978). They have been named the Loa and Kea trends, after the two tallest volcanoes on the Island of Hawai’i, Mauna Loa and Mauna Kea.
Additionally the volume flux of Hawaiian eruptive products has increased by roughly 650% along the Hawaiian Ridge and additional 375% for the formation of the Hawaiian Islands (Wessel, 2016). This implies that the Hawaiian mantle plume has strengthened over time, which is rather unusual for mantle plumes Tree (2016).
In the following article I want to introduce you two the two views that are currently discussed in the scientific community to explain these rather strange occurrences.
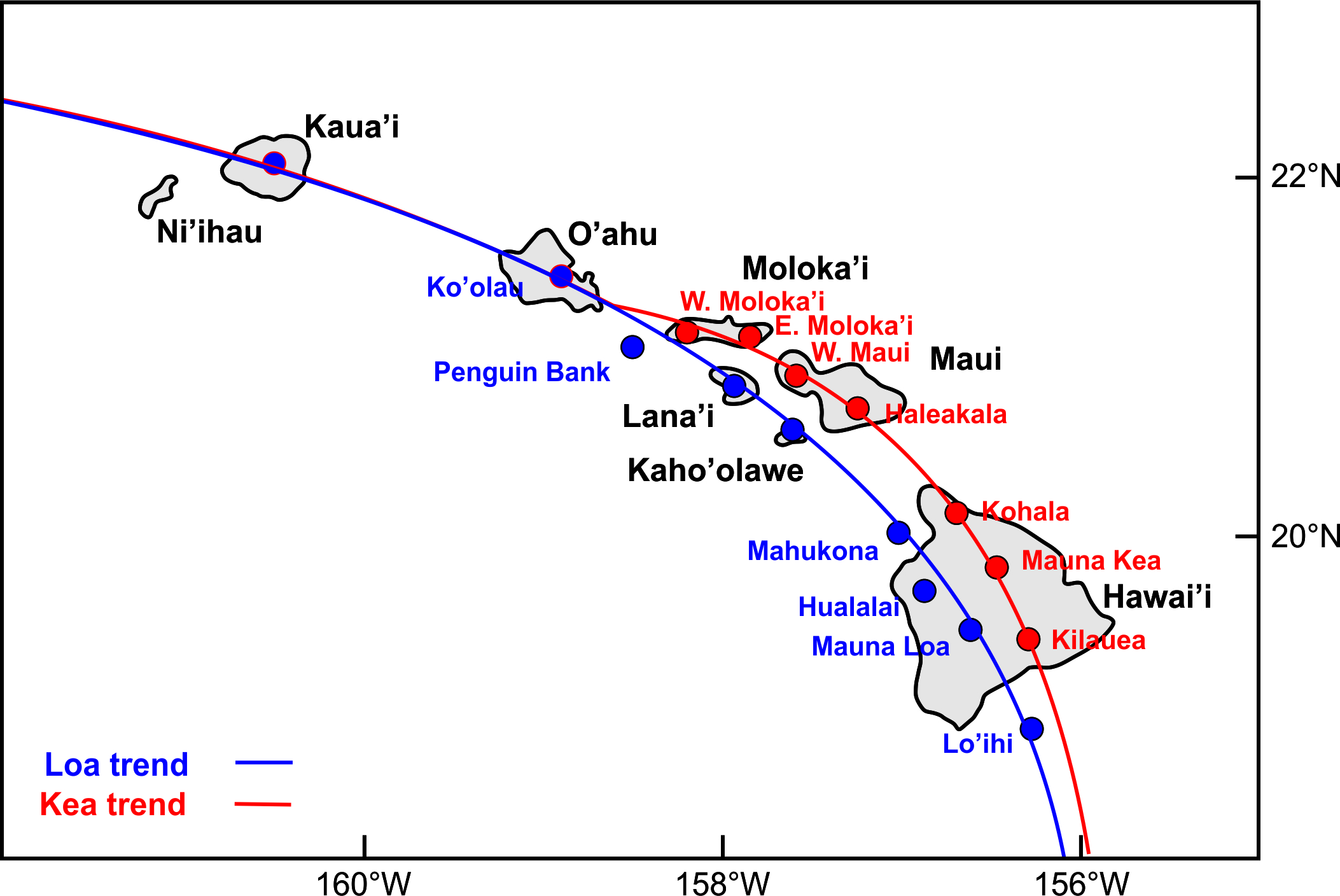
Map of recent Hawaiian volcanism, highlighting the two volcanic chains.
Modified after Jones et al. (2017).
Option 1: The plume as a window into deep mantle structures
Mantle plumes bring materials to the surface that have originated deep in the mantle. Geochemical studies show that the Earth’s mantle is chemically heterogeneous at scales ranging between centimeters to thousands of kilometers (Sun and McDonough, 1989). Radiogenic isotope ratios can be used to identify those different mantle source regions.
The two volcanic trends at Hawai’i show distinct differences in their Pb-isotope ratios. This was fist observed by Abouchami et al. (2005) and vastly extended by Weis et al. (2011). If these geochemical differences are a direct influence of the underlying mantle plume, than this mantle plume needs to display azimuthally asymmetric compositional zoning (Farnetani and Hofmann, 2010). Seismological studies and mantle flow models show that, if the mantle plume is projected down to the Core-Mantle boundary, it overlaps the edge of the large low-shear-velocity province (LLSVP) beneath the Pacific (To et al., 2011).
The LLSVP is thousands of kilometers wide, sharp-sided (~400-600 km thick), chemically heterogeneous, long-lived piles of anomalously seismically slow material in the lower mantle below the Pacific Ocean (Garnero et al., 2016). It is suggested to represent either a remnant of a primordial magma ocean (Williams et al., 2015) or accumulations of subduction recycled crustal material (Weis et al., 2011).
When transferred to the surface, the Kea side of the Hawaiian volcano chain overlies ambient Pacific mantle and the Loa side overlies the Pacific LLSVP. Continuous sampling of these two qualitatively different types of mantle could have resulted in the observed surface differences between the parallel volcano chains. The steep sides of the tall LLSVP would effectively anchor the plume (Jellinek and Manga, 2004), ensuring continuous melt generation at the edge. The bilateral asymmetry of melt will then stay intact during ascend and even remain through the strong tilting the top of the plume undergoes due to the moving Pacific Plate (Weis et al., 2011).
Recent studies by Harrison et al. (2017) add onto the research of Weis et al. (2011) and have shown that the Loa component is not observed in the Emperor Seamounts and only emerged recently.
The final model of Harrison et al. (2017) states that initiation of the Hawaiian plume started close to the margin of the Pacific LLSVP. Here, it firstly only sampled material from the surrounding Pacific deep mantle. The surface impingement of this intial plume phase then subsequently formed the Emperor Seamount chain. During its lifespan the plume slowly drifted towards the edge of the LLSVP, where it eventually anchored itself. During this time, the material from the LLSVP slowly got entrained into the rising plume. The quantities of this entrainment rose with time to its current state, causing increased magma flux.
If the Loa component is predominantly explained by the presence of subduction recycled material (Weis et al., 2011), the source can be considered to be more fusible and its introduction into the melting process has been proposed to be linked to the increased magma influx in Hawaiian volcanoes (Harrison et al., 2017).
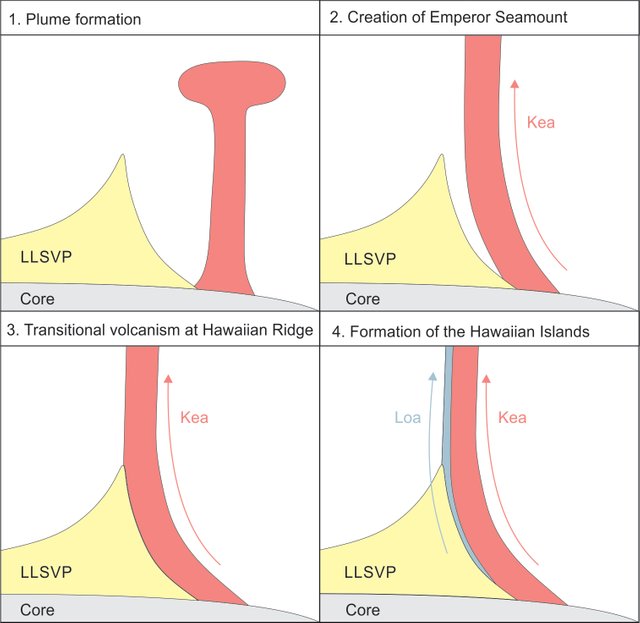
Evolution of the Hawaiian mantle plume at the Core-Mantle boundary. Initiation of the plume close to the LLSVP. Wandering towards the LLSVP over time and anchoring at its steep flanks. Additional melting of LLSVP component transported upwards in the ascending plume, causing the geochemical differences observed in the double volcano chain.
Modified after Harrison et al. (2017).
Option 2: Melt generated heterogeneities in an ascending, tilted plume
Opposed to the idea of hotspots preserving separate upwelling material from the deep mantle, Jones at al. (2016) concluded that it is premature to assume that the heterogeneous structure and composition of Earth’s lowermost mantle can be directly observed on the Earth’s surface. They show, using numerical modelling, that chemical density contrasts will prevent bilaterally zoned plumes from developing. In the two proposed compositions of the LLSVP, oceanic crust and primitive Fe-rich mantle material, this contrast would be greater than 2%. The authors therefore state that if deep mantle LLSVP is interpreted to be a dense, stable and long-lived thermochemical pile then bilateral plume scenario on the origin of bilateral asymmetry recorded in Hawaiian and other Pacific hotspot lavas is invalid.
But Jones et al. (2016) also acknowledge that the thermo-chemical structure of deep-mantle LLSVP is strongly debated. Recent studies show that the seismic characteristics of the LLSVP can be explained by thermal differences alone and that chemical differences are largely a passive component of lowermost mantle dynamics (Davies et al., 2015). In such a scenario, bilateral plumes would ascend and allow a geochemical observation of deep mantel structures at the Earth’s surface.
Following the doubts seeded, Jones et al. (2017) proposed an alternative three-part model for Hawaiian double-track volcanism:
- At first, the moving Pacific plate above the mantle plume causes the plume to strongly tilt in the low-viscosity upper mantle, thus leading to a separation between high-and low-pressure melt regions, both facing towards the same direction.
- Secondly, recent azimuthal change in Pacific plate motion (more towards the north) leads to these two melt regions to become geographically separated and forming simultaneous double-track volcanism.
- At last, melting of secondary pyroxenite creates geochemically distinct lavas. It is created from the previous reaction of eclogite melt with surrounding peridotite in the ascending plume and has a higher solidus temperature than the surrounding peridotite. Due to this fact it dominates the low pressure melt region. This melt region lies beneath the Loa-track and is the reason for the systematic geochemical differences between Loa- and Kea-type lavas.
With their model, Jones et al. (2017) address why geographically distinct double-volcano chains have only recently occurred. They state that gradual change of plate movement caused the observed opening of the double chain 4.2 million years ago at Kō’olau and reached a maximum value about 2 million years ago. After this the mantle plume beneath the Pacific plate started to adjust its tilt to the new direction of plate movement and the spatial distance between the two volcano chains is gradually becoming smaller. This first increasing and after 2 million years decreasing pattern is also visible in the isotopic signatures reported by Weis et al. (2011).
The fact that the Loa component is not visible in any other volcanoes of the Hawaiian Ridge is explained by Jones et al. (2017) by the recent rise of mantle temperature, which is the cause for the increased magma flux at Hawai’i. These higher temperatures enable the proposed secondary pyroxenite to form and to then create the observable geochemical differences.
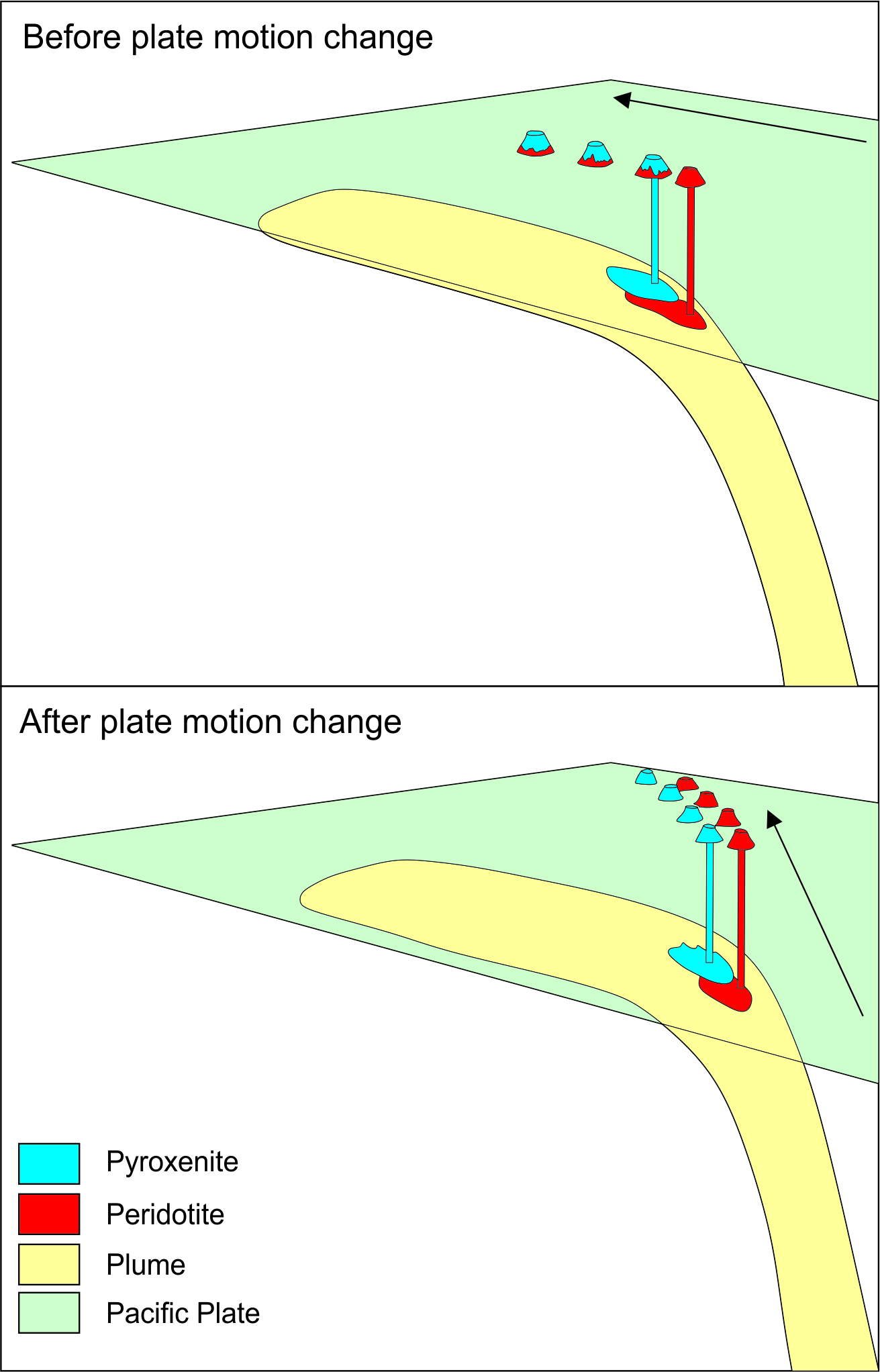
Geographic change in observed melt ascension before and after plate movement changes.
Modified after Jones et al. (2017).
Summary
Hawai’i, although intensively studied, is surprising scientists to date. The still ongoing debate about its exact origins show how little we really know about our own planet.
A double volcano chain, having started to form around 5 million years ago, is clearly observable. In addition to their geographically different positions, they also display different geochemical signatures. Two very different models have been proposed to explain their creation. In the first, the sampling of different material in the deep mantle gets transported through the mantle in a bilaterally zoned plume, impinging the crust and erupting two different lava compositions. In the second model, the melting of secondary pyroxenite a shallow environment causes the observed geochemical differences. The geographical differences of the two chains, are purely a result of a motion change of the Pacific plate.
I am sure, as more research is conducted, that this discussion will move on and I am looking forward to watch its progression.
Previous Posts
Hawaiian volcanoes - Part 1: Introduction
Hawaiian volcanoes - Part 2: The Hawaiian Hotspot
Hawaiian volcanoes - Part 3: A growing volcano
Hawaiian volcanoes - Part 4: The Rejuvenation Stage
Hawaiian volcanoes - Part 5: Evolution to Atolls and Seamounts
Hawaiian volcanoes - Part 6: Mythology vs Geology
Hawaiian volcanoes - Part 7: Recent eruptions
Hawaiian volcanoes - Part 8: Volcano Monitoring
Hawaiian volcanoes - Part 9: Predicting Eruptions
Hawaiian volcanoes - Part 10: Volcanic Air Pollution
Hawaiian volcanoes - Part 11: Volcanoes falling apart… from Landslides and Tsunamis
Hawaiian volcanoes - Part 12: Benefits of Volcanoes
References
- Abouchami, W., Hofmann, A.W., Galer, S.J.G., Frey, F.A., Eisele, J., Feigenson, M. (2005). Lead isotopes reveal bilateral asymmetry and vertical continuity in the Hawaiian mantle plume. Nature 43 4, 851-856.
- Davies, d.R., Goes, S., Lau, H.C.P. (2015). Thermally dominated deep mantle LLSVPs: a review. In: Khan, A., Deschamps, F. (Eds), the Earth’s Heterogeneous Mantle. Springer International Publishing, 441-477.
- Farnetani, C.G. and Hofmann, A.W. (2010). Dynamics and internal structure of the Hawaiian plume. Earth and Planetary Science Letters 295, 231-240.
- Garnero, E.J., McNamara, A.K., Shim, S.-H. (2016). Continent-xized anomalous zones with low seismic velocity at the base of the Earth’s mantle. Nature Geoscience 9. 481-489.
- Harrison, L.N., Weis, Dominique, W., Garcia, M.O. (2017). The link between Hawaiian mantle plume composition, magmatic flux, and deep mantle geodynamics. Earth and Planetary Science Letters 463, 298-309.
- Jellinek, A. and Manga, M. (2004). Links between long-lived hot spots, mantle plumes, D’’ and plate tectonics. Reviews of Geophysics 42.
- Jones, T. D., Davies, D. R., Campbell, I. H., Wilson, C. R. & Kramer, S. C. (2016). Do mantle plumes preserve the heterogeneous structure of their deep-mantle source? Earth Planetary Science Letter 434, 10–17.
- Jones, T.D., Davies, D.R., Campbell, I.H., Iaffaldano, G., Yaxley, G., Kramer, S.C., Wilson, C.R. (2017). The concurrent emergence and causes of double volcanic hotspot tracks on the Pacific plate. Nature.
- Sun, S. and McDonough, W. (1989). Chemical and isotopic systematics of oceanic basalts: Implication for mantle composition and processes. In: Magmatism in the Ocean Basins. Eds. Saunders, A.D. and Norry, M.J. Geological Society of London Special Publication 42, 313-345.
- Tatsumoto, M. (1978). Isotopic composition of lead in oceanic basalt and its implication to mantle evolution. Earth and Planetary Science Letters 38, 63-87.
To, A., Fukao, Y., Tsuboi, S. (2011). Evidence fo a thick and localized ultra low shear velocity zone at the base of the mantle beneath the central Pacific. Physics of the Earth and Planetary Interiors 184, 119-133. - Tree, J.P. (2016). Mantle Potential Temperatures of 4.5 to 47 Ma Hawaiian Volcanoes Using Olivine Thermometry: Implication for Melt flux Variations. M.Sc thesis, University of Hawai’i at Manoa, Honolulu, 126 p.
- Weis, D., Garcia, M.O., Thodes, J.M., Jellinek. M., Scoates, S. (2011). Role of the deep mantle in generating the compositional asymmetry of the Hawaiian mantle plume. Nature Geoscience 4, 831-838
- Wessel, P. (2016). Regional-residual separation of bathymetry and revised estimates of Hawaii plume flux. Journal of Geophysical Research 113.
- Williams, C.D., Li, M., McNamara, A.K., Garnero, E.J., van Soest, M.C. (2015). Episodic entrainment of deep primordial mantle material into ocean island basalts, Nature Communications 6, 1-7.
You received a 80.0% upvote since you are a member of geopolis and wrote in the category of "geopolis".
To read more about us and what we do, click here.
https://steemit.com/geopolis/@geopolis/geopolis-the-community-for-global-sciences-update-4
Awesome topic and presentation @fooflauschig! The presentation is great as well with outstanding referencing worthy of a publication!
Exactly the thing we should be pushing towards in the scientific community! The Jones et al., 2017 paper is pretty cool too. Keep up the awesome work and look forward to your next postings.
Thank you :)