THE CHEMISTRY AND THE CONCRETE
Why Chemistry Interests FOR THE CONCRETE?
Chemistry and Concrete
The first principle to understand is that, in a broad sense, concrete is thermodynamically unstable. When the cement paste is exposed to the atmosphere, it begins to deteriorate, which is a form of chemical corrosion. The paste will react to exposure to acid rain or to the normal amount of carbon dioxide in the air. This reaction causes the surfaces to flake and carbonate, and then, the calcium silicate is hydrated to give the concrete strength that will convert it again to calcium carbonate, silica gel, and aluminum gel.
This is an aspect of chemistry, but chemistry is not only thermodynamic, it is also kinetic. In other words, concrete has the potential to change, but how quickly will this happen? Concrete made carefully with the right materials in the right proportions can last for many hundreds, and even thousands of years. An example of this is the Pantheon in Rome, built with a Roman Pozzolanic Cement, which has lasted for more than 2000 years.
When Joseph Aspdin patented his Portland cement in 1824, it was titled An Improvement in the Ways of Producing Artificial Stone. Concrete was considered as an artificial stone that has the appearance, strength, and durability of the real thing. Some of the early concrete structures that were made with the Aspdin cement are still standing today, none destroyed by wear and tear.
The important aspect is that concrete (the cement paste system that binds the particles in the body of an artificial stone) is inherently reactive and, given its particular structure and exposure conditions, that reactivity will result in excellent or poor durability. . In terms, short or long, it is chemistry that makes the difference in the permanence of concrete.
Examples of typical exposure agents that affect durability are:
- Moisture and water of napas.
- Cycles of temperature.
- Thawing salts.
- Marine environment.
- Carbon dioxide and acid air pollutants (NO2 and SO2).
Depending on the composition of the concrete and the exposure conditions, several chemical reactions can deteriorate the concrete. Sometimes, however, the enemy is not some external element, but rather, the seeds of destruction that they can innocently contain within the concrete itself.
Moisture, or water, play the most important role in the setting, the development of resistance, and the eventual deterioration of the concrete. Portland cement hardens due to a chemical process called hydration.
This means that silicate and aluminate minerals in portland cement react and combine with water to produce the "tail" that holds the aggregates together called concrete. Portland cement is only one type of hydraulic cement, and it should be noted that ASTM C 1157-00, the performance specification for cements, is called "Standard Performance Specification for Hydraulic Cement", and includes portland cements and mixture.
As Duff Abrams emphasized in his seminar paper "Design of Concrete Mixtures", however, which he presented at the December Meeting of 1918 of the Portland Cement Association and then published in Bulletin 1 of the Lewis Institute, the water - cement relationship (a / c) dictates the strength of the concrete. Although the size and granulometry of the aggregate and the amount of cement influence the amount of water required to produce a workable mixture, it controls the strength of the concrete in a mixture. Therefore, you should use the smallest amount of water that produces a plastic concrete, or workable. Water can, depending on the quality and nature of the aggregate used and the history of concrete curing, trigger such other deleterious events as alkali aggregate reactivity (ASR) or delayed ettringite formation (DEF). Water can also act as a means of transport for potentially aggressive species, such as sulfates, to enter the system.
Temperature affects the speed of chemical reactions, and a general rule of thumb is that a chemical reaction rate doubles for each 10 ° C increase in temperature. Then, the temperature influences the setting speed and the hardening. Curing concrete above certain critical temperatures can lead to expansion and cracking associated with DEF.
Several external environmental factors can initiate destructive chemical reactions in concrete, particularly concrete with more open porosity (due to a high a / c ratio). Some of these factors include deicing salts containing chloride used to treat roads in winter, spraying salts or exposure to seawater tides, and soils containing sulphates or napa water.
Chlorides can slowly diffuse into the concrete and, in the presence of moisture and oxygen, will initiate the corrosion of the armor. Oxidation of iron to produce iron oxide is a chemical process that generates a large volume of oxidation product that not only structurally weakens the metal, but also produces internal localized pressure that can cause severe cracking of the concrete coating. Once cracking has started, more concrete surface is exposed to a subsequent chemical attack.
Chemical reactions of Cement
Portland cement contains calcium silicates and aluminates formed by a sequence of thermal and chemical processes, including the decomposition of limestone, the reaction with other quarry materials such as clay, iron ore, and sand; the partial fusion of these components, and gives rise to the formation of hard, rounded nodules called clinker. All this occurs at a temperature of 1450 ° C in a rotary kiln of a cement plant. After cooling, the clinker is milled together with approximately 5% gypsum (calcium sulfate dihydrate) to a flour-like fineness, producing the final product, portland cement (Kosmatka and Panarese, 1994).
The present knowledge of the chemical composition of portland cement and what happens to it when it is mixed with water was first revealed in 1887 by the French chemist Henry Le Chatelier (1905). In his doctoral thesis, he correctly identified the important minerals of cement such as tricalcium silicate, dicalcium silicate, and tricalcium aluminate.
In 1915, scientists at the Geophysical Laboratory in Washington DC, were studying the phase relationships in high temperatures of the ternary system CaO-SiO2 - Al2O3. Among the mineral phases investigated were, of course, the tricalcium silicate, the dicalcium silicate, and the tricalcium aluminate. In the process of publishing the phase diagram in the form of a triangle, certain simplified abbreviations were invented for the chemical compositions of each mineral phase. For example, the tricalcium silicate, Ca3SiO2, could also be written as the combined sequence of the two oxides, such as 3CaO.SiO2. The researchers, Rankin and Wright, then used the abbreviated notation of CaO = C, SiO2 = S, and Al2O3 = A. Accordingly, 3 CaO.SiO2 could be written as C3S. Similarly, the dicalcium silicate, 2 CaO.SiO2, was transformed into C2S, and the tricalcium aluminate, 3CaO.Al2O3 was transformed into C3A (Bogue and Steinour, 1961).
This notation was so convenient and useful that, in future publications during the following years, a similar notation was introduced for the other oxides. Then, Fe2O3 = F, MgO = M; H2O = H; Na2O = N and K2O = K. The additional problem of the S of sulfur trioxide SO3 was solved simply by indicating it with S bar. Other names for cement minerals or their hydrates concurrently entered into the language and are commonly used today. For approximately 100 years, the silicate minerals tricalcium and dicalcium, in their impure composition as they crystallize in the clinker have been called alita and belite, respectively. Others are named by their mineralogical equivalent name, such as periclase for MgO crystals in cement, portlandite for calcium hydroxide (CH in abbreviated notation) and ettringite for hydrated calcium silicoaluminate (C3A.SiO2.32H). The formula for ettringite in cement chemistry notation seems to be complicated, but is simplified compared to a conventional chemical formula (Ca5 [al (OH) 3] 24 H2O. (3SO2). (2 H2O).
Normal hydration reactions
The fastest reaction that occurs when mixing cement and water is the hydration of tricalcium aluminate (C3A). Only he himself, C3A and water will quickly form tricalcium aluminate hydrates such as C4AH13 and C2AH8.
This can happen so quickly that the concrete can become thick after a few minutes and become completely unbalanced due to the heat emitted. This condition is called false setting. In the nineteenth century, when cement developed resistance slowly because it was ground into large particles and reacted imperfectly, false setting was not a problem. Eventually, with the introduction of rotary kilns, a more scientific proportion of the components of the raw materials and a much finer grinding of the clinker, the addition of gypsum (CSH2) to the cement eliminated the problems of false setting.
Chemically C3A, gypsum and water would form a protective coating of hydrated calcium sulfoaluminate (ettringite) on the exposed surfaces of tricalcium aluminate that would remain for several hours.
The hydration of C3A is reactivated as the beginning of setting begins, consuming the sulfate and forming more ettringite. If portland cement is of the ASTM Type I type, however, it probably contains more than 8% C3A, which is a much higher percentage than the sulfate present.
When all the sulphate has been combined as ettringite, the excess of C3A continues to be hydrated, and then begins to remove sulfate from some of the ettringite (trisulfate) to form another stable compound called calcium sulfoaluminate monosulfate, C3ACSH12 (Eqs 1 and 2) .
A fourth important mineral in cement, the iron-containing ferrite phase, or tetracalcium aluminoferrite (C4AF) is also hydrated, albeit much more slowly, to form chemically similar compounds trisulfate and monosulfate, in which iron (Fe2O3) replaces a portion of aluminum (Al2O3) (Steniour, 1958).
Cement chemists generically call these the Aft (aluminate-ferrite-trisubstituted) and AFm (aluminate-ferret-monosulfate) phases, respectively.
C3A + 3CSH2 + 26 H ® C6AS3H32 (ettringite) (1)
2 C3A + C6AS3H32 + 4 H ® 3 C4ASH32 (2)
(monosulfoaluminate)
he development of the main strength of the concrete, however, results from the hydration of the calcium silicate phases (C3S and C2S). Both calcium silicates are combined with water to form hydrated gel-like calcium silicate, or C-S-H (Eqs 3 and 4)
2 C3S + 6 H ® -S-H + 3 CH (3)
2 C2S + 4 H ® C-S-H + CH (4)
Chemistry and cracking
Cracks in concrete can develop for a physical reason such as shrinkage by drying or mechanical loading. Local chemical reactions in concrete, however, can also cause expansion, internal pressure development, and then cracking. Concrete is a brittle material and can therefore be expanded only to a limited degree before cracking.
In broad terms, the observed expansion is equal to the sum of the widths of the fissures. If it is not possible to determine the cause of the expansion and cracking by the appearance of the fissure pattern on the surface of the concrete, the interior concrete samples should be examined microscopically or chemically, to determine the cause of the internal expansion.
In field concrete exposed to aggressive elements, there are two basic modes of expansion:
- The aggregate can be expanded in relation to the cement paste, and
- The cement paste can be expanded in relation to the aggregate.
It follows from physical considerations that, in a system composed of particles that expand in a matrix, radiant fissures are formed from the particles in the matrix. The expansion of the particles is a hardened paste, such as the aggregate particles that undergo the RRAA, causes the particles to crack and the fissure to extend outward into the surrounding paste. A cracking of the particle, when it expands from the surface, is really an almost common experience.
When the ice cubes are dropped in a drink and the sound of a familiar crackling is heard - this is the sound of ice heating up on the outside, expanding and then fissuring. The expansion in the surface causes the inner part of the particle to be under tensile stress, and fissures from the inside to the outside.
The shrinkage of cement paste is a common phenomenon related to hydration, and from a cracking point of view, it is equivalent to the expansion of aggregate particles. What would happen if the paste really expands in relation to the aggregate particles?
When the cement paste expands in relation to the aggregate particles, as it is in the DEF, spaces are opened around the particles. This concept is really a bit counterintuitive, in that one might expect the pasta that expands to actually break the particle rather than create a space around it. This is similar to the question of whether a hole that has been drilled through a piece of metal would become smaller or larger in diameter as the metal heats up and expands.
One way to understand the phenomenon is to consider the concrete constituted by aggregate that does not expand, and to carry out the following imaginary experience. First, assume that the concrete, including the paste and aggregate, expands by 20%, in all directions. There is no distortion, no cracking, and concrete is somehow more widespread. Then, since the aggregate particles do not expand, contract them to their original size. What happened? The particles then scatter around the holes and, in addition, these spaces are proportional to the size of the particles.
Alkali reactivity - silica
The chemical reaction AA occurs between the highly alkaline solution of the pores (very high pH) and the reactive siliceous portions of some aggregate particles. The large amount of hydroxyl ions (OH-) present in the pore solution, due to the highly alkaline concentration (sodium and potassium), dissolves the reactive silica on the surfaces of the aggregate to form an alkaline silica gel. Although any form of silica can react with alkali hydroxides in theory, it is siliceous rocks such as opal, chert, and glassy volcanic materials that appear to be the most reactive.
The reactive siliceous aggregates will form a silica-alkali gel starting at the surface of the aggregate and moving inward. The tensile forces produced during the reaction cause the aggregate particles and the surrounding paste to crack. In several cases, the fissures will interconnect and lead to the weakening of the concrete. The weakening is due only to fissures; the paste between the fissures maintains its composition and resistance.
The densest polycrystalline rocks, such as granites, will react more slowly. The chemical reaction will occur in those heterogeneous areas of the edges of the grains. In such cases, only a minimal degree of reaction may be necessary to cause cracking.
In the deterioration by RRAA, given that each internal fracture in the concrete creates an empty space, the alkali - arid reaction causes the volume increase (Helmuth, 1993). The visual evidence resulting from the reaction is the "cracking map" observed on the surface of the concrete.
Conclusion
Going back to the original question: Why does chemistry matter?
The answer can be summarized as follows: Chemistry matters because the composition of the concrete and its behavior are based on a variety of chemical reactions that vary from the original setting and hardening of the constituents of the cement to the desired engineering properties.
The durability of concrete depends on the chemical processes that both cement and aggregates develop, curing conditions and exposure to a variety of environmental effects. The chemical reactions that occur during the hydration of the clinker minerals determine the microstructure of the concrete.
Hardened concrete is chemically reactive. Therefore, it is essential to design suitable concrete mixtures and erect structures in a way to adequately control or compensate for chemical reactivity.
To hear the speech version of this post click the play image.
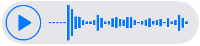
Brought to you by @tts. If you find it useful please consider upvote this reply.
Please Help me with your upvote!!
@thepajaro es increíble la cantidad de cosas que hay detrás de algo tan común como el concreto. La química lo es todo! Saludos de parte de la familia de @anytrades :)
Así es...y esto es solo desde el punto de vista quimico, de este tema todavia hay mucha tela que cortar...saludos