The bible is back and the new version 4 is taking care of the Higgs boson
Of course I will not talk about religion, even if I used the word ‘bible’ in the title of my post. And I will not talk about Halloween as well. This was the non-serious post of last Sunday.
Now it is indeed time to go back to serious things: particle physics. And I will use this post to discuss various small points here and there :)
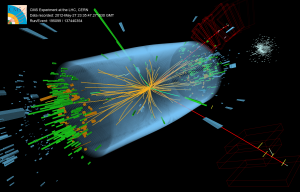
[image credit: the CMS collaboration]
Last week, on October 25th, a handbook on Higgs physics has been released after more than a year of hard work. This article, which has been posted as a CERN Yellow Report, summarizes the state-of-the-art knowledge on the Higgs boson and its properties. It will serve as the bible on Higgs physics for what concerns the second run of the LHC.
And now you know why I choose the title of this post!
This handbook consists of the most complete review of the knowledge on the Higgs boson up to now, and includes the major expectation of the high-energy physics community for the next few years in terms of new phenomena.
It collects the work of 374 physicists and counts 868 pages (which is why there are so many authors). Releasing it as a CERN yellow report is a way to maintain the knowledge free access. This report has been posted on the arxiv and will not be submitted to any journal.
I will refer below to this article as the Yellow Report 4, or the YR4, as it is the fourth yellow report on Higgs physics. The list can be found here.
And for completeness, its title is Deciphering the nature of the Higgs sector.
BUT WHAT IS IN THERE?
The starting point of this work is given by the image below:
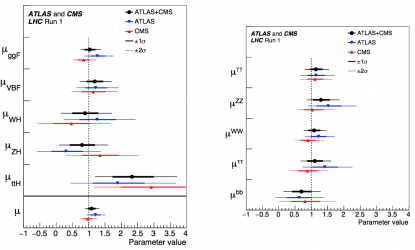
[image credits: The YR4]
These two figures show the best fit results for the production modes (left) and the decay modes (right) of the Higgs boson in the light of LHC Run I data. The value 1 means that data matches the Standard Model expectation and a value different from 1 would mean that we have either an excess or a deficit. And thus something new!
As shown there, the ATLAS and CMS combined results (the black circles) show a remarkable agreement with the Standard Model. But there is still room for new phenomena as the error bars are still large enough for that.
The second run of the LHC has started in the meantime, in 2015, with an increased collision energy. The rates at which a collision would yield a final state containing a Higgs boson have increased. This increases reaches in general a factor of 2 to 4, depending on the production mode, so that we will have at our disposal much more Higgs events to scrutinize its properties within the next few years. As a result, it is important to have a document like the YR4 to be well prepared.
GETTING ORGANIZED
The work reported in the YR4 focuses on different aspects of Higgs physics, which is why 374 physicists joined their forces. The achievement of writing up such a document has thus required a huge organization in order to have the work correctly done and efficiently managed. I will try to quickly describe this organization below.
As I already said elsewhere, this relies on a tree and each leave double-checks what is going on beyond it.
The different topics that have been covered have been classified into topics covered by three working groups. Each of these working groups is managed by 4 or 5 responsible people named conveners who have taken care of having all contributions written in due time and reviewed.
Within a given working group, the ensemble of covered topics is subdivided into subcategories managed by subconveners (in charge of the writing of a small set of chapters of the YR4), and the writeup of each chapter has been coordinated by one person, who was communicating both with the subconveners and the large number of people interested by the chapter subject.
After the subconveners were all happy with the chapters they were in charge, the final text was reviewed by the conveners of the different working groups and finally by the 9 editors of the reports who where in charge of the final YR4.
1 - STANDARD MODEL PREDICTIONS
If we want to observe deviations from the Standard Model, we need very precise predictions for the Standard Model (to be sure that a deviation is really a deviation). The first part of the report therefore concerns Standard Model precision calculations for Higgs boson production and decay in the Standard Model
An example of these is given in the figure below. It depicts the rate for producing a Higgs boson via the fusion of two gluons (the main Higgs boson production mode) computed at different levels of accuracy.
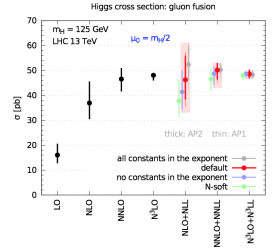
[image credits: The YR4]
The x-axis is very simple to understand. LO means leading order, NLO next-to-leading order and so on. The more ‘N’ we have, the more precise is the calculation. It is easy to notice that an easy-to-compute LO prediction for the Higgs boson production rate would be off by a lot with respect to the really predicted value.
In parallel, we can track down specific contributions and compute them at a higher level of accuracy (this is what the NLL, NNLL and N3LL labels are, without entering into details).
More complicated and precise calculations actually allow for a better control of the rate and of the uncertainties.
The calculation associated to the rightmost point is the most precise particle physics calculation performed of all time!
2 - THE HIGGS PROPERTIES
The second big point addressed in the report concerns the properties of the Higgs boson, and the room that is left for deviations from the Standard Model or for new phenomena.
Several frameworks exist on how to use the data in the best possible way to extract information about new physics (a synonym for physics beyond the Standard Model). Among them, one very important one (and one that I actually like very much) is the effective field theory framework. I have already discussed it this in this older post by the way.
This works very simply. The starting-point assumption is that there are new particles to be observed, but they are too heavy to be accessible within the reach of the current experiments. As a result, the only way to find their tracks is an indirect way: those new particles induce tiny deviations in the properties of the Standard Model particles that can be noticed.
Particle physics relies on symmetries, and those symmetries allow us to model those deviations in a very-well defined manner. The form of the deviations is fixed, and only their magnitude or strength is to be bound by data.
3 - PHYSICS BEYOND THE STANDARD MODEL
The last part of the YR4 concerns specific new physics theories that can be used as benchmarks for the exploration of a non-standard Higgs sector. This includes typical scenarios (like supersymmetric scenarios) featuring multiple Higgs bosons and exotic Higgs boson decays.
Just to give a few words about it, a supersymmetric theory is a theory with a larger symmetry than the one of the Standard Model. It associates with each Standard Model particle a partner, named a superpartner. Standard Model fermions receive hence sfermion superpartners, whilst the gauge the Higgs bosons get gaugino and Higssino superpartners. A particle and its superpartner have a different bosonic/fermionic nature (see here for some info about that).
In all of these models, the theory contains more than one single Higgs boson and there are thus new particles to find, observe and study. For instance, a possible exotic Higgs decay is given in the figure below.
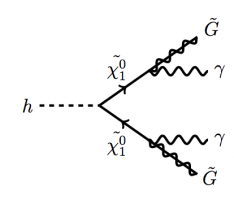
[image credits: The YR4]
The Higgs boson (h) decays here into a pair of neutralinos. A neutralino is a supersymmetric particle that is a partner of the gauge and Higgs bosons, or an admixture of the higgsinos and gauginos. In the process described by the figure, the neutralinos decay each into a gravitino, whose properties can be cooked so that the gravitino is a candidate for explaining the problematics of dark matter, and a photon.
Studies of non standard Higgs boson demand many calculations that have actually been achieved for the report.
SUMMARY
The Higgs YR4 collects the work of many physicists and is the reference on Higgs physics for the next few years.
If interested, do not hesitate to give it a look. Maybe the introductions of each chapter is a good start to get an idea what this big report is about and what is inside. Do not hesitate to ask questions, maybe on the #steemSTEM chat.
I sometimes wonder is there is ever an end to the number of particles or if it just get smaller and smaller :D
I am not sure to get the comment. If you can provide more details, maybe I can try to provide an answer :)
I think he means; is we keep finding things smaller and smaller as our ability to see them gets better. He is wondering if there is an end, like the smallest thing fathomable or if we keep finding things inside of things inside of smaller things and smaller forever.
@lennstar is that what u mean?
All the particles in the Standard Model are elementary, which means that they do not have any substructure. Moreover, quantum mechanics connects the uncertainties on positions (related to sizes) and momenta (related to energies). This means that we have some practical limitations here: having a physical state with a very precisely defined small size means that we are loosing control on the rest.
I hope this gives an answer, at least partially :)
basically. I also did not mean it in a strictly science way. Of course there has to be an end somewhere. It is just that once we thought the atom is the smallest thing. Then we thought the electron. Then... so where does this end? We will probably need two more millenias to find out.
Ah I see. Check my answer to @rafaelzauner. This should answer your question... and the answer is: "we don't know" :)
Which is why particle physics is exciting those days (at least for me)!
Not only for you!
I am currently running about 40 work units from LHC@home and 10 more from vLHC on my computers.
that's great! Thanks for contributing to our field :)
Included in Steemprentice Spotlight :)
Thanks a lot!
lennstar hits on a interesting point. Here is a different way of visually looking at your question. The Planck Length is quasi the smallest possible unit to be had. Quarks are extremely small and never observed alone. You need 3-quarks to build a proton or neutron, depending on the quarks. Now the relationship between the size of the Planck Length and the Quark is like comparing the size of the quark to the observable Universe, I read somewhere. So there seems to be lots of possibilities left going down in scale I imagine if that comparison is right.
Concerning the Planck scale (~ 1e18 GeV), we do not know. What we only start to know more about is the electroweak scale (~ 100 GeV) and the puzzle is however still not fully resolved (the Higgs boson is only one piece of it).
As you can see from the above numbers, there are many orders of magnitude between the electroweak and the Planck scales (which has by the way lead to the infamous hierarchy problem). Why such a hierarchy? We do not know. As a result and since this sounds very unnatural, we expect new phenomena to occur between these two scales. But maybe there is just nothing. This is why beyond the Standard Model physics is important today. We need to be prepared regarding any possible option that we will (or will not) discover.
Only (future) data will (hopefully) tell us :)
PS: the three-quark picture does not hold at the LHC. The proton is a much more complex dynamical object (you can check my last particle physics post for instance).