Drosophila
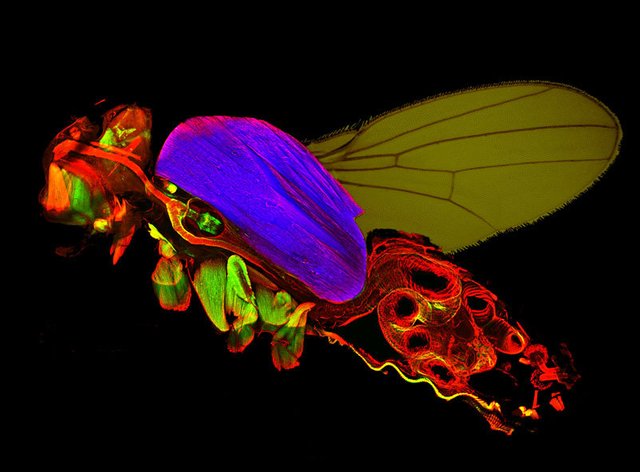
By examining the development of the Drosophila fruit fly, we can explore how specific genes influence pattern formation.
Many aspects of Drosophila development are unique, but the general mechanisms are similar to those involved in the development of many animals, including vertebrates. We begin with a discussion of how the segmented construction of insects illustrates the basic principles of pattern formation and then discusses the original experiments that identified the genes responsible for Drosophila pattern formation. Then describing one set of Drosophila genes that act as master switches in pattern formation, as part of a regulatory cascade of interacting genes. We conclude today by discussing homeotic genes, which act as localized master switches triggering the development of specific structures, and that illustrate deep genetic similarities in developmental mechanisms among very different types of organisms.
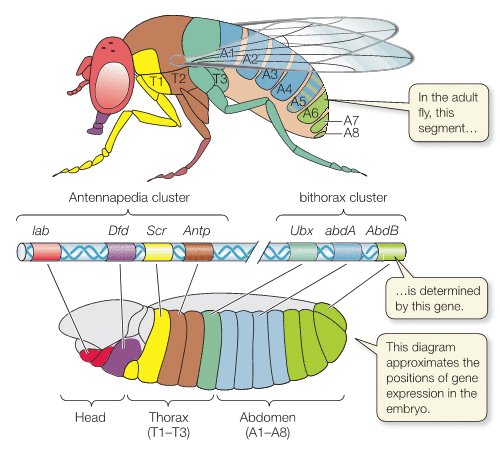
The Drosophila fruit fly is an ideal study organism for several reasons, including its role as an example of pattern formation.
Fruit flies, like all insects, are highly segmented, their bodies have an obviously modular construction. Segmentation is most obvious in the larval stage, which is a juvenile period, such as the caterpillar stage in the development of butterflies. The larval form of a fruit fly is a small grub, which has obvious rings marking the segments along its anterior posterior (head-to-tail) axis. When the larva later develops into an adult, these segments specialize into different regions of the head, the thorax, and the abdomen. In this way, segmentation establishes the functional differences that develop along the anterior-posterior axis.
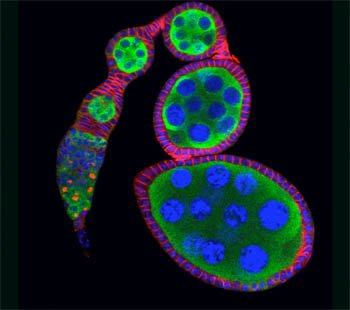
The early development of Drosophila differs from that of other organisms we have discussed.
In Drosophila, cytokinesis the final separation of daughter cells does not occur during the first 10 or so rounds of initial nuclear replication. The result is one cell with many nuclei (a multinucleated cell). As these nuclei continue to divide, they line up along the walls of the cell, leaving no nuclei in the center. Suddenly at about the 13th round of division cell membranes partition all the nuclei into very small individual cells. The result is a hollow oval of small cells that is approximately the same size as the original zygote. This hollow ball of cells is called a blastula, just as in sea urchins and frogs. As the Drosophila blastula undergoes gastrulation and the embryo develops, body segments begin to appear, with obvious differences between the head and the tail ends. These segments at first look similar, but even adjacent segments will develop in different ways.
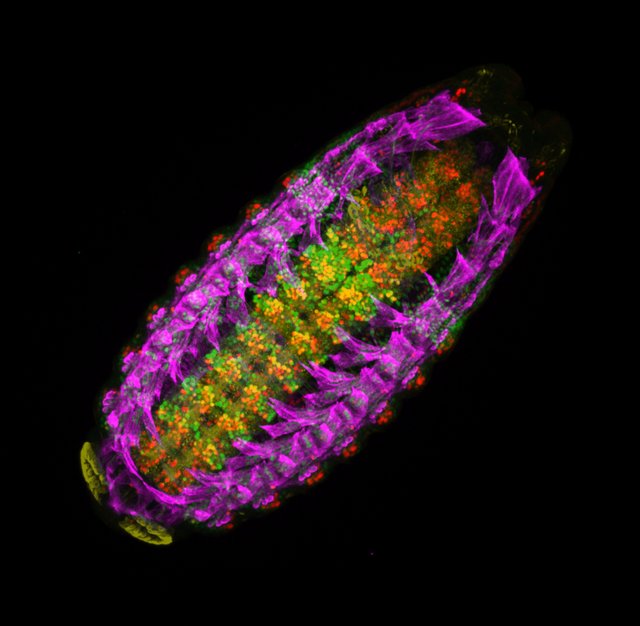
This description of Drosophila development raises two questions concerning pattern formation.
First, how does a segmented structure arise from an unsegmented one?
Second, how do segments at different points along the anterior posterior axis develop into the different structures appropriate for their positions?
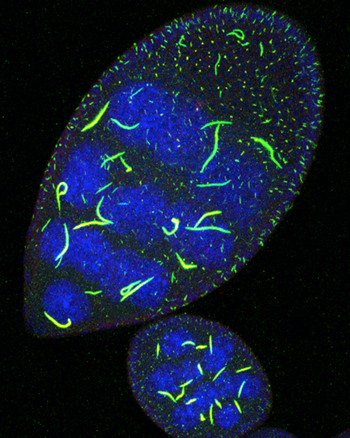
The answer to both these questions involves the differential expression of genes.
Many different genes control segmentation and the emergence of the anterior-posterior axis in a Drosophila embryo. The majority of these genes are regulatory genes their products are transcription factors that turn on and off the expression of other genes. These genes are expressed sequentially, which builds patterns from the original asymmetry of the maternal determinants in the Drosophila egg.
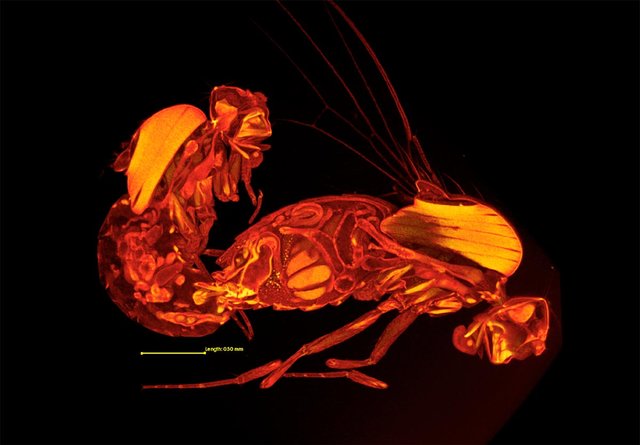
Experiments in the 1970s identified genes responsible for pattern formation in Drosophila.
Two developmental geneticists, Christiane Nüsslein-Volhard and Eric Wieschaus, set out to identify genes involved in pattern formation in Drosophila by exposing them to mutagens and examining which mutations appeared to affect pattern formation. This project was ambitious and difficult, but Nüsslein-Volhard and Wieschaus were able to identify more than 1,000 genes that affect development, about 120 of which specifically affect pattern formation. One of the first mutations the researchers described was of a gene they named bicoid, which means “two-tailed.” Bicoid mutant larvae lack heads they are essentially two tails attached to each other at the middle. When Nüsslein-Volhard and Wieschaus performed genetic crosses with individuals carrying the mutant gene, they found that bicoid larvae display a pattern of inheritance called maternal effect inheritance. This means that the phenotype of the offspring depends entirely on the genotype of the mother, rather than on whether the larva inherits the mutant gene itself.
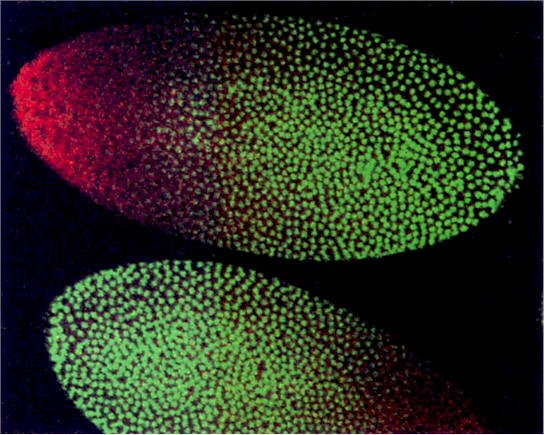
Maternal effect inheritance is caused by cytoplasmic determinants added to the egg by the mother.
The protein produced by the bicoid gene is normally distributed asymmetrically along what will become the anterior-posterior axis of the larva. Because the cells that produce bicoid mRNA come into contact with only one end of the egg, there is a much higher concentration of the mRNA on that end than on the other end of the egg. If bicoid mRNA is injected into the head end of an egg with the bicoid mutation, the embryo develops a normal head. Similarly, if bicoid mRNA is injected into the tail end of a normal egg, the egg develops into a two-headed larva.
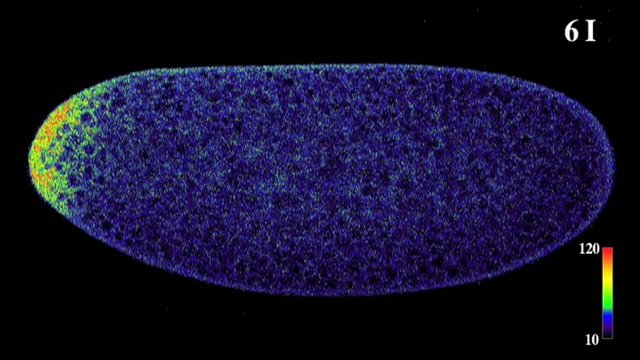
The bicoid gene performs its role in pattern formation by coding for a transcription factor that influences the expression of other genes.
The protein produced by the bicoid gene activates other genes that ultimately involve the development of head structures. The end of the embryo with a high concentration of bicoid protein becomes the head, and the end with a low concentration of bicoid becomes the tail. Bicoid, however, is not the only “switch” that turns on genes involved in making a Drosophila head. Instead, bicoid and other maternal determinants control a cascading sequence of other genes, which in turn, produce other transcription factors. A single factor such as the asymmetric distribution of the bicoid protein coordinates the activities of the many genes involved in constructing a head by activating a cascade of additional switches, each of which specifies and controls the expression of other genes. In a developing
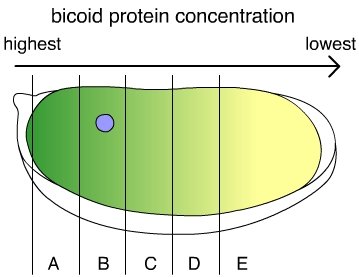
Drosophila embryo, the initial gradient of bicoid establishes the basic segmentation pattern by regulating the activity of segmentation genes, which come in three classes: gap genes, pair-rule genes, and segment polarity genes.
Bicoid and other maternal determinants regulate the activity of the gap genes, which organize large areas into basic subdivisions along the anterior-posterior axis, setting up a slightly more complex grid of signals along this axis. F. The gene products of the gap genes, in turn, regulate the expression of pair-rule genes, which divide the embryo into pairs of segments. Pairrule gene products are also transcription factors that regulate the activity of segment polarity genes—which themselves produce transcription factors. This cascade of transcription factors produces an increasingly refined and complex pattern of gene expression.
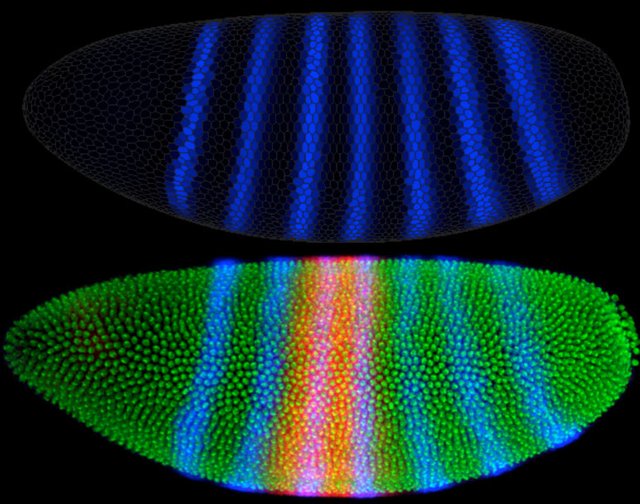
The pair-rule gene called the even-skipped gene, or eve for short, illustrates this cascade.
The eve gene’s protein is normally expressed in seven discrete bands along the length of the embryo. The eve gene itself is preceded by a very complex regulatory region that contains seven different regulatory sequences. The regulatory sequences can be activated independently, and each is responsible for the production of one discrete band of the eve gene’s protein product along the axis of the developing embryo.
At the stage in development where pair-rule genes are beginning to be expressed, there is a very complex set of gradients of transcription factors along the axis of the embryo. At any place along the embryo where one of eve’s regulatory sequences finds the right combination of proteins, eve is turned on.
The second band of eve expression (eve stripe 2) corresponds to the regulatory region called the stripe 2 module. One simplified model suggests that this module includes binding sites for two regulatory proteins that activate transcription and binding sites for two regulatory proteins that repress it.
The relative concentrations of these four proteins determine whether or not transcription occurs, and this combination of concentrations occurs only at one narrow region along the embryo’s head-to-tail axis. In this way, a regulatory cascade produces gradients of several transcription factors that together regulate gene expression in a highly localized fashion.
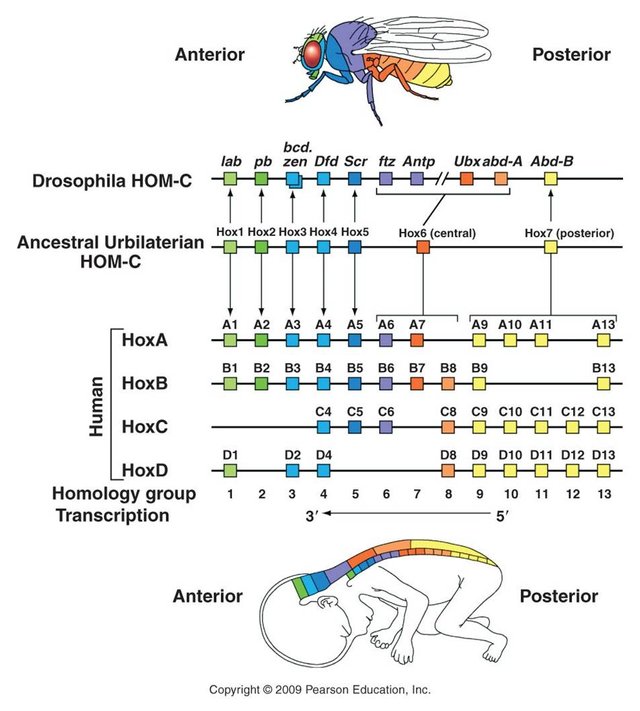
The differentiation of relatively uniform segments into diverse parts depending on their location along the head-to-tail axis is mediated by the activity of another set of genes called homeotic genes.
Once segmentation genes have established body segments, homeotic genes specify the types of appendages and other structures that each segment will form. Mutations in homeotic genes produce extremely bizarre morphological transformations, because they cause the development of structures in the wrong places on the animal, such as the development of a leg on a head segment that would ordinarily have produced an antenna. Like segmentation genes, homeotic genes code for transcription factors that, in turn, regulate the expression of many other genes. Therefore, homeotic genes are best thought of as master switches that control the expression of many other genes in a localized way. The Hox genes in vertebrates are remarkably similar in function and location to the homeotic genes found in Drosophila—so close that a mutant fruit fly can receive the appropriate Hox gene from a chicken and develop properly. This is remarkable considering that insects and vertebrates have not had a common ancestor for hundreds of millions of years. The similarity of homeotic and Hox genes illustrates a deep commonality in the basic developmental genetic pathways of animals and suggests that a basic genetic “toolbox” evolved very early and has been used in similar ways to create the varied body plans of all organisms.
Sources: 1
Image Sources:
https://www.mpg.de/630623/zoom-1368460380.jpeg
http://www.stephenandrewtaylor.net/images/sadava_19_19_FULL.jpg
http://www.stowers.org/sites/default/files/images/profiles/Xie/Xie_web_pict1.jpg
http://thenode.biologists.com/wp-content/uploads/2011/06/E1-copy.jpg
http://www.drosophila-images.org/images-2015/CTP-synthase-filaments-small.png
http://cdn.phys.org/newman/gfx/news/hires/2015/3dscansofmat.jpg
http://www.bionalogy.com/images/bicoid02.jpg
https://i.ytimg.com/vi/O91xQWdqz7M/maxresdefault.jpg
http://web01.cabeard.k12.in.us/science/APBiology/bc_campbell_biology_7/media/objects/2560/2621894/quiz/haq2114q.jpg
https://ittakes30.files.wordpress.com/2010/06/drosophila-eve.jpg
http://eweb.furman.edu/~wworthen/bio111/hox1.jpg
Bird [Giphy](https://giphy.com/gifs/blue-5lDik5jPpRw2Y)
Thanks #steemit for Donating!
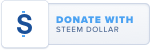
BTC: 1JQWNxziArfaCtxsP3tUQ4VSTBY9jresmt
Zcash: t1fKGLRuwDM4KUTfE9f8nso3dkB9Ew1KUod
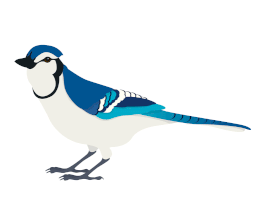
This post has been linked to from another place on Steem.
Learn more about and upvote to support linkback bot v0.5. Flag this comment if you don't want the bot to continue posting linkbacks for your posts.
Built by @ontofractal