Genotoxicity. [eng]
It is as bad as it sounds. How can chemical agents cause DNA damages, what are the consequences and how do our cells react to it?
CC0, pixabay
Intro
You might have noticed that my last full post was a while ago. The reason is that at the moment we are in the middle of a very labor-intensive animal study, which is strictly following Murphy's law. So I don't really have time and energy to write.
I am responsible for an experiment that has to be repeated daily for several weeks, even on weekends (yay!): the so-called "Comet Assay."
So last week, I had the spontaneous and glorious idea to just write a post about it, because it is definitely one of the cooler methods I work with. So far, so good... But then I quickly realized that it would probably not be interesting enough without explaining a few basics in advance.
Thus, I first wrote another post. It is part of the unofficial series that I call "fundamentals of toxicology", and it's about Genotoxicity.
This is the English adaption, you can find the German original here.
By the way, the cool and trendy toxicologist phrases the word differently: "Genotox." I would like to say the full expression every time... but I really don't have time for that!
"Oh, damn, wrong format... Yeah, yeah, okay, it'll be a full post.
The DNA
Most of you will have a rough idea of what the deoxyribonucleic acid (DNA) is. Anyway, allow me a short crash course to really bring everyone to a basic level we can work with:
The DNA is like the hard disk of life, a macromolecule consisting of a phosphate chain (the "backbone") to which 4 different bases (Adenine, Guanine, Cytosine, Thymine) are bound at regular intervals via a deoxyribose (a sugar) molecule. Such a chain of "nucleotides" (1 phophate + 1 sugar + 1 base), is called "strand". A DNA strand does not remain alone, but binds to a second one, whereby one A pairs with one T and one C with one G, which means that the second strand is exactly complementary to the first. This results in double strands, which then form the famous helix structure:
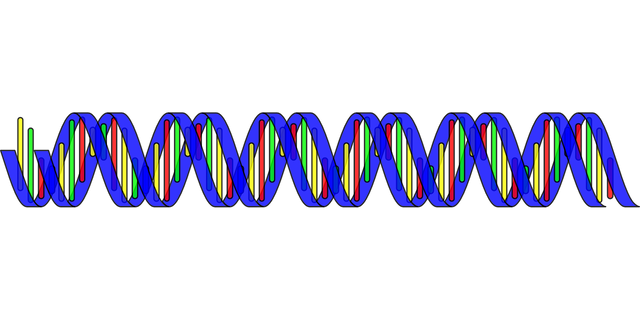
Double-strand helix, schematic, colored, simple. CC0,pixabay
The unique sequence of these base pairs is the biological code, a program that tells our cells what they have to do and when they have to doo it (or more precisely: which protein they have to produce when in what quantity).
This works by first transcribing the DNA, which is closely packed together within the cell as a chromosome in the cell nucleus, into a related molecule, the RNA. The RNA is transported from the cell nucleus into the cytosol and serves as a "blueprint" for the proteins that are produced ("expressed") there.
A piece of DNA coding for a particular protein is called a gene.
Genotox
As the name tells, a substance is genotoxic if it is "toxic to genes" (please note the incredible creativity we scientists are capeable of when introducing terms), i.e. it is capable of damaging the DNA. This can happen in several ways:
Strand breaks
Nomen est omen. When a DNA molecule is broken off or cleaved, it is referred to as a _strand break. A distinction is made between a defect in only one ("single strand break" - SSB) or both strands ("double strand break - DSB").
This can happen in different ways:
Some substances are intercalating in the double helix and thus hinder enzymes at work, which can lead to the DNA breaking off at one point. Others interact directly with enzymes that unravel the DNA (e.g.topoisomerases) and thus lead to deliberately generated gaps in the DNA no longer being closed or the torsional stress becoming too high.
But the most serious possibility is certainly if the phosphate backbone is attacked and cleaved directly, as it is e.g. caused by electromagnetic rays (UV light, gamma rays).
However it happens, the result of strand breaks is "fragmented" DNA.
Base oxidation
Not only the backbone, but also DNA bases can be the target of a chemical attack. Oxidizing substances can modify the bases at different points in such a way that they can no longer be read correctly, which can lead to errors during protein synthesis, but also when copying the DNA.
This happens mainly during what is known as "oxidative stress" (I have blogged about this before), i.e. when the cell's own antioxidants are no longer sufficient to keep radicals and other reactive oxygen species under control.
Substances that can cause oxidative stress at a sufficiently high dosage (!) are numerous like sandcorns at the sea (yay, quoting the bible again!) and it is difficult for me to pick out individual examples... alcohol, red meat, various mould toxins,... just name it.
adduct formation
A particularly serious damage to DNA is very similar to oxidative damage, with one decisive difference: during base oxidation, the bases undergo minimal changes. During adduct formation, big molecules are attached to the bases. This causes two problems: first, a different type of DNA repair is required, which works much worse (more on this below), and second, such "bulky adducts" completely block enzymes that want to read or copy the DNA.
Examples for adduct-forming compounds are benz[a]pyrene that is produced when meat is charred over an open flame, or the mycotoxin aflatoxin B1 (I reported). And of course this:
Tar and soot particles also contain adduct-forming agents. Fun fact: when testing our own blood for DNA damages in a preliminary study, our smoking technician held the high score. CC0,Pixabay
Cross-links
Another possibility is the formation of cross-links between the DNA strands, like the chemotherapeutic agent cisplatin is causing.
Consequences of DNA damage
Imagine someone scratching the hard drive of your PC. It will stop working correctly. The same happens to the cell if the DNA is damaged. Proteins are no longer produced correctly and can no longer carry out the roles assigned to them. The DNA is copied and incorrectly passed on to new cells - so-called mutations occur.
The problem with mutations is not that they would cause an armada of X-Men to step out of the dark - that only happens in modern fairy tales. In fact, mutations increase the probability that cells mutate into cancer cells. This might happen when the mutations affect genes that code for proteins whose function is to control cell growth, cell death and division (so-called "oncogenes").
Well-known examples of such proteins are p21, which is involved in stopping the cell cycle after DNA damage is detected, or of course the "guardian of the genome", the p53, which "makes the decision" whether damage should be repaired or controlled cell death is preferable.
Genotoxic substances are therefore usually classified as carcinogenic and carry the corresponding warning sign when you buy them (Which you probably can't. But I can. Never mess with your next-door toxicologist!):
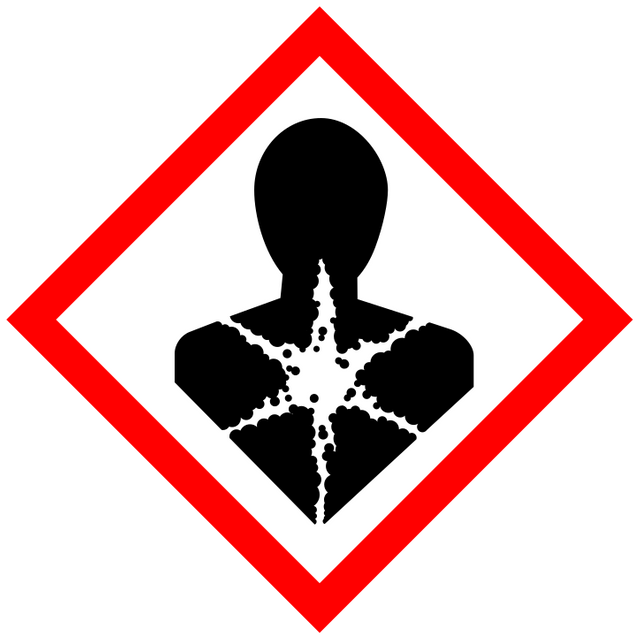
That means for us lab rats: put on your lab coat, gloves on, work carefully, dispose in hazardous waste. Or at least that would mean it if the world were full of responsible people... (image is public domain.)
DNA repair and apoptosis
Now, we humans are constantly exposed to environmental influences that damage our DNA. Nobody lives without ever seeing the sun, breathing in smoke, doing sports or eating something. In fact, we can also detect a certain basic damage to DNA in healthy people. Approx. 0.2-0.5% of our DNA in blood cells (which get a not-so-healthy dose of all compounds we take up) is always damaged. Not exactly no damage at all.
However, if cells were to give in and mutate due to such damage, highly developed life - that requires an extent of genome stability - would be impossible. That we can exist is due to the cellular ability to repair damaged DNA.
Cells of higher organisms produce various proteins whose function is to recognise damage to the DNA.
If damage is detected, a signalling cascade is triggered that stops the cell cycle (the recurring process of cell division) in order to give other enzymes the time to repair the DNA before it is duplicated. Only a successful duplication of damaged DNA would be considered a mutation, as a new cell would be formed whose genetic code differs from that of the parent cell (which can then also no longer be recognized and repaired).
Depending on the type of damage, different repair mechanisms take effect, which run at different speeds and with different accuracy:
- If individual bases are oxidatively damaged, the Base Excision Repair (BER) is used, in which a damaged base is cut out and replaced by an intact base. This process is relatively simple, fast and virtually error-free.
- If there are large adducts at the base, the BER machine cannot dock and it is necessary to cut out a large piece (about 20-30 nucleotides) from the DNA and replace it - the so-called Nucleotide Excision Repair (NER), which works significantly slower and more incorrectly than the BER.
- If single strand breaks occur, the gap in the strand is closed, with the complementary strand providing information on which bases are to be installed where.
- Double-strand breaks pose a particular problem, although we distinguish between two szenarios: If the information about the original DNA code is still available ("homologous repair") or not (non-homologogeous end joining, NHEJ). @suesa once described these repair processes in a post, and since I don't want to reinvent the wheel, I save myself the details... Only this much: Especially the NHEJ only works to a limited extent and with some probability leads to mutations.
It can therefore be said that our cells tolerate a certain mutation rate for their own survival, which is indispensable for the functioning of the organism, but naturally entails a certain risk of cancer.
If the DNA repair is successful, the cell cycle is released again and everything continues as before. However, if no improvement occurs after a certain time, the cell commits suicide in order not to endanger the organism as a whole, a process known as apoptosis.
Summarized:
DNA damage caused by environmental influences (radiation, chemicals, food,...) is detected and repaired by cells. If the repair is not possible, the cells undergo apoptosis. However, both the recognition and the repair of the damage (depending on their type) is not possible without errors, which can lead to mutations that increase the overall cancer risk.
But why test it?
For us toxicologists, genotoxic substances are naturally interesting and alarming. However, in order to regulate them legally and thus improve public health, the effect must first be demonstrated experimentally.
But there is also interest from the other direction: There is the so-called "health claim" of the EU, which entitles companies to market scientifically proven positive health aspects of their products accordingly. One effect that many of them are targeting is the protection against DNA damage. And you have to be able to prove that too.
For measuring DNA damage, we have a repertoire of methods, both in cell culture and in vivo. We can measure mutation rates in bacteria or determine apoptosis induction based on the activity of certain proteins, we can show the generation of genes that react to DNA damage... Or we can measure DNA strand breaks directly - for example with the Comet Assay.
But that's what the next post is about.
Sources statement: I hope you enjoyed the little trip into the world of our DNA. Most of the information I have extracted from my head, as it concerns my field as a scientist. Excuse me for not starting to browse the Internet for supplementary links right now. I would do it, but I really don't have time for this!
This post has been voted on by the steemstem curation team and voting trail.
There is more to SteemSTEM than just writing posts, check here for some more tips on being a community member. You can also join our discord here to get to know the rest of the community!
Hi @sco!
Your post was upvoted by utopian.io in cooperation with steemstem - supporting knowledge, innovation and technological advancement on the Steem Blockchain.
Contribute to Open Source with utopian.io
Learn how to contribute on our website and join the new open source economy.
Want to chat? Join the Utopian Community on Discord https://discord.gg/h52nFrV