The New Optical Technology For Accelerating Particles : Economic And Compact
Two of the publications have recently reported the first experimental realization of a new technique for accelerating charged particles-laser acceleration over dielectric structures. It captivates not only a strong accelerating field, but also its cheapness, completely optical design, compactness and simplicity of scaling. The practical implementation of such an "accelerator-on-a-chip" will dramatically reduce the cost and increase the availability of accelerators for applied research.
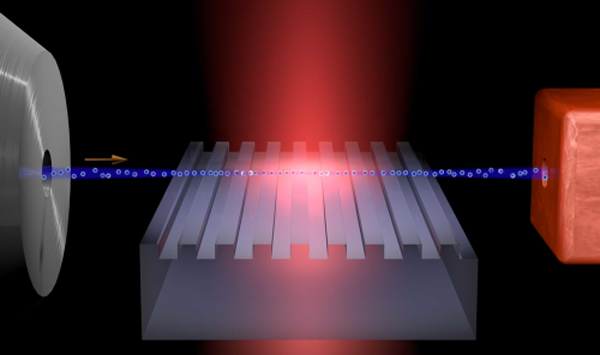
Fig. 1. Diagram of the operation of a dielectric laser accelerator. On the quartz glass with periodic grooves of submicron size, a laser beam shines, and along the surface, perpendicular to the grooves and the ray, an electron beam starts. The evanescent light wave traveling along the surface picks up electrons and accelerates them with its field.
Difficulties in accelerator physics
Particle accelerators are needed not only for physicists, but also for ordinary people. Of the tens of thousands of accelerators that now exist in the world, only about a hundred are working for their intended purpose, to study the microcosm. All the rest are used to solve applied problems in biology, in materials science, in medicine and even, oddly enough, for studying the history of the Ancient World (that's just one example). A brief listing of these applications can be found, for example, in the brochure Accelerators and Beams, Tools for discovery and innovation (PDF, 7 MB).
The main task of the accelerator is to accelerate electrons, protons and other particles to the required energies. Of course, energy is not the only characteristic of a particle beam; it is usually required that it be narrow, intense, well focused, monochromatic, with a good longitudinal profile, etc. But the primary task is precisely acceleration. Accelerate the charged particles with a longitudinal electric field, and the stronger the field, the more effective is the acceleration. The conversion of the field into energy is elementary. If an electric field of 1 megavolt per meter (MV / m) is created inside the installation, then the accelerating gradient is the same 1 MeV / m, that is, on each meter of the path, the electron or proton energy is increased by 1 megaelectronvolt (MeV). If you want to accelerate an electron to an energy of 100 GeV, be kind enough to provide a 100-kilometer stretch with such a gradient, or figure out how to increase it.
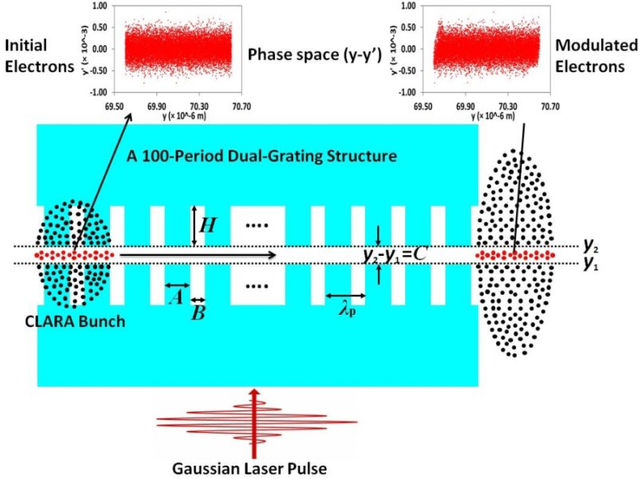
Fig. 2, Particle accelerator; Schematic of an electron bunch travelling through the optimum structure to interact with a laser pulse.
Accelerating field intensity in megavolts and even tens of megavolts per meter modern technology still achieves. Usually a powerful standing electromagnetic wave is excited inside a specially constructed superconducting chamber of complex shape, which pushes particles passing through it (you can check your skills of particle acceleration in the flash game LHC Game). However, a gradient of more than a few tens of MeV / m in such chambers can not be obtained - the metal simply does not withstand a too strong field, the chamber breaks down. That is why a linear electron-positron collider for an energy of the order of 1 TeV will be long, several tens of kilometers, and, as a consequence, quite expensive.
Another option is to make the accelerator not linear, but cyclic, that is, circular (see the device of a typical accelerator on an interactive poster). The particles in it constantly circulate inside the annular tube, and do not travel the entire distance only once. Then the accelerator section can be set modest, but the energy can be increased, it would seem, without restrictions - because the particles will fly it millions of times per second. Unfortunately, there is another problem. Particles with too much energy are difficult to hold on the annular path. On the linear section - please, but as soon as the particle needs to be turned, it must be applied to it. And this is again achieved due to the external field - this time the magnetic field inside the rotary magnet. Since it is limited, it is necessary to turn the particles gradually, that is, to increase the turning radius. Therefore, high-energy ring accelerators, such as the Large Hadron Collider, also turn out to be huge.
In the case of cyclic electron accelerators, an additional problem arises: electrons, when rotating, emit electromagnetic waves and lose energy. Therefore, the accelerator section should, first of all, compensate for energy losses on each turn, and only then increase the energy. And when only one maintenance of energy requires spending hundreds of megawatts (!), Further increasing it becomes simply unprofitable. And for a linear trajectory, there are no such problems.
It turns out that in both types of accelerator there is a natural restriction on particle energy, and it arises because we still do not know how to create and keep sufficiently strong electric and magnetic fields. No conventional, now debugged, accelerating technology can cope with this problem.
Fortunately, for the vast majority of application accelerators this is not a problem. The energy required there is small, of the order of hundreds of MeV, they can also be obtained on a plant several meters in size. But other technical problems remain, from the highly technological process of manufacturing accelerator sections to the complex infrastructure and high energy consumption. And compact such accelerators you will not name: under them in any case it is necessary to allocate the whole building. About a desktop, and even more portable accelerator can only dream of.
Attempts to break the vicious circle
The only way to dramatically reduce the size of accelerators and reduce the cost of their production is to find a new particle acceleration technology that would increase the accelerating gradient even to hundreds of MeV / m. And there is hope for this. The fact is that in principle the field of tension in many gigawolts per meter is easy to obtain; the main difficulty is how to keep it, because such a field will cause breakdown of metal walls.
Two methods are well known, as this difficulty is bypassed: these are laser and laser-plasma accelerators. These technologies have long been heard, see a video story, a lecture with numerous animations, a selection of popular materials about it, a detailed overview article, as well as an article, a news item, and a task on Elements. In laser accelerators, the metal foil is irradiated by a super-power laser pulse (peak power of the order of petawatt), which literally "blows" electrons out of the foil. Laser-plasma technology uses not metal structures, but cells with plasma; if the plasma is removed from the state of equilibrium, it can generate fields as many as hundreds of gigawolts per meter. Of course, the plasma will not keep such a field, but it is not necessary. It is enough to create it inside the bubble, which will fly along with a bunch of particles and accelerate it on the entire length of the plasma chamber. This technology is not a fantasy for a long time, it has already been successfully demonstrated in the experiment. Gradients of tens of GeV / m have already been reached, that is, one thousand (!) Times more than with traditional technologies, albeit in a very short section, a few millimeters long.
These promising technologies have, however, disadvantages. The first is problems with scalability. Laser accelerators generally have nothing to say: there, acceleration is obtained only once when burning one sheet of foil. In laser-plasma, huge fields are demonstrated while inside a small chamber no larger than a few centimeters in size. To accelerate at higher energies, it is required to dock many of these chambers to each other and synchronize the formation of the plasma bubble in all of them. This task remains unresolved, although the first experiments on the docking of the two chambers have already been carried out.
Another obvious problem is the behavior of the beam of accelerated particles. After all, the beam has to not only fly through the plasma itself, but also constantly pass through the walls of the chambers. Whether this is compatible with the required beam parameters and their intensity is a complicated question; in any case, it is necessary to puzzle over how not to spoil the beam with acceleration.
And finally, there is a problem with the cost. Even if it is possible to create, say, a compact proton laser accelerator for burning out cancerous tumors, it will still use a high-power laser, and this is a very expensive device.
However, to the credit of laser-plasma accelerators, it must be said that their potential is far from exhausted. A few months ago, a circuit was described and numerical simulation of electron acceleration in a periodic plasma structure was carried out. Accelerating gradients there are quite sky-high, a lot TeV / m. If this can be realized, then Higgs bosons can be born in a desktop accelerator. However, the path from the idea to the experimental realization is long, so these proposals lie, so far, rather in the desired than the actual.
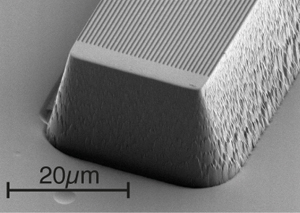
Fig. 3. Electron micrograph of a transparent bar made of quartz glass with periodic grooves etched on it.
New acceleration technology
In the current situation with traditional and laser-plasma accelerators, one more technique, the dielectric laser accelerator, seems very attractive. Without promising huge accelerating gradients, this scheme captivates with its simplicity, scalability, compactness and cheapness. It was proposed not so long ago, and until now everything was limited only to theoretical studies of this type of accelerator. But now the situation has changed: the other day in the journals Nature and Physical Review Letters came out two articles, which reported on the first successful implementation of this method. The electron acceleration achieved in these studies is so far negligible, but there has never been a pursuit of high efficiency-these experiments have only successfully proved that the method works. It is already clear how easily to improve all the parameters of the beams.
Let us briefly outline the essence of dielectric laser acceleration using the example of the article in Phys. Rev. Lett. In a tiny sample of a transparent dielectric (for example, quartz glass), long parallel grooves are etched with a period of a micron fraction (Fig. 3). A phase diffraction grating is obtained, but with a very small period. A laser beam with a wavelength slightly greater than the grating period is passed through the glass through the glass. And right above this structure, parallel to the surface of the glass, a compact electronic clot flies by. He feels only the laser beam - directed, notice, perpendicular to the movement of electrons! - but precisely this laser light accelerates it (Fig. 1).
Due to what happens here acceleration? Light with linear polarization, perpendicular to the grooves, induces polarization on them. Therefore, in a vacuum directly above the surface, there exists an oscillating periodic electric field (Fig. 4). If the period of the structure is too small, then this field is held near the surface, like a kind of "virtual" light, and can not fly up. This is the so-called near light field, or the evanescent wave (see the problem on a similar topic). It can be represented as a set of electromagnetic waves that run along the surface of the glass, but perpendicular to the grooves; this motion is shown in Fig. 4 in the form of consecutive "frames" of the field state. The speed of these waves is easily adjusted by selecting the wavelength of light. Now the important point - the electric field in this wave is also directed along the surface, parallel to the direction of the wave movement. There are ideal conditions for particle acceleration: if the electron cluster moves at the same speed as the wave, then it simply picks it up and carries it forward, simultaneously dispersing its electric field.
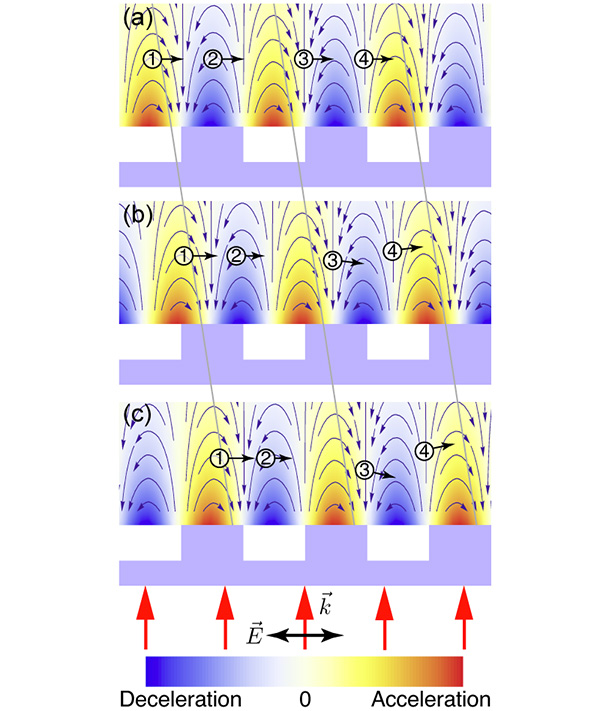
Fig. 4. Principle of acceleration in a dielectric laser accelerator. Laser light coming from below creates an electric field, alternating in time and space, parallel to the surface. Three consecutive images show the configuration of the field at three subsequent time points, divided by a quarter of the period of the oscillation of the light wave. Flying over the surface and falling into this field, the charged particles will accelerate (particle 1), slow down (particle 2), or diverge (sides 3 and 4) depending on their location.
In another article published in Nature, the system is only slightly more complicated. There, instead of one glass with grooves, two were used, exposed by the teeth opposite each other and separated by a gap in the fraction of micron (Figure 5, left). The whole structure was approximately half a millimeter in length and width (Figure 5, right). The laser beam also shone perpendicular to the surface, along which an electron bunch flew in the gap and was accelerated by an electric field.
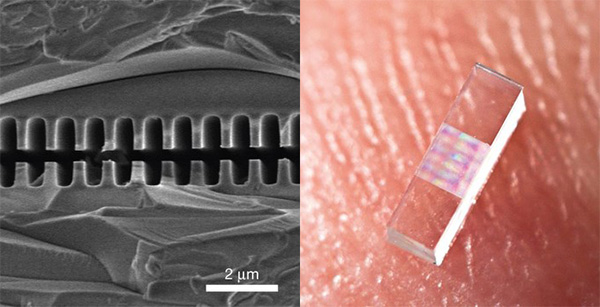
Fig. 5. Electron micrograph of the gap between the two grilles (left) and the whole structure at the tip of the finger (right).
The accelerating gradient achieved in the first article is not too impressive: 25 MeV / m (which, incidentally, is already comparable with the records of traditional accelerator technologies). However, such a modest result was obtained only because the authors of the article set themselves a more complicated task than usual - to disperse electrons that were initially non-relativistic. Relativistic electrons are much more efficient in accelerating, and in the second article we are talking about a gradient up to 300 MeV / m. Apparently, this gradient can be increased several times more.
Due to what in this method it is possible to receive an accelerating gradient an order of magnitude more than in traditional technologies? Yes, simply due to the fact that it uses not a metal, but a dielectric. The metal responds more sensitively to a strong electric field, since it contains free electrons. Therefore, those fields that will cause a breakdown in the metal chamber, dielectric still holds. Studies have shown that such quartz glass structures hold a field of at least 9 GV / m, which means that the gradient above 1 GeV / m in such an accelerator seems quite real.
Advantages of the new technology
The above number, of course, greatly exceeds the accelerating gradients in modern accelerators, but it is far from the records of laser-plasma accelerators. However, the dielectric accelerator has several impressive advantages at once, which make it a serious competitor.
First, its manufacture and management is incomparably simpler than for traditional or laser-plasma accelerators. The necessary structures are easily and mass-produced on existing technological lines, for example, on those where microchips are produced. To make a single glass with several structures moving in succession is simpler (Figure 6, left). The laser in both works was the most common, commercially available, and not at all some kind of superpower.
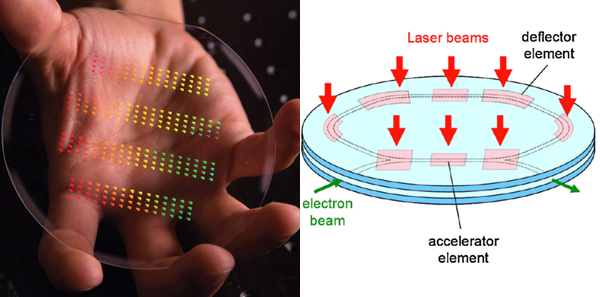
Fig. 6. To the left: a quartz plate with hundreds of necessary dielectric structures; right: the conventional scheme of the "accelerator-on-chip" installation in which all the elements of the cyclic accelerator are made in the form of structures in a solid plate and are controlled by laser pulses.
Secondly, the dielectric accelerator perfectly scales. Make many such glasses, as in Fig. 6, and install them in one vacuum chamber with a neat alignment in height, apparently, it is easy. Phase synchronization is also easy to achieve: you just have to start each structure in this chain with a single laser pulse. There is, however, a certain subtlety, how to start with nonrelativistic electrons and end up ultrarelativistic, but, as the first article shows, it is completely surmountable. Therefore, compact installations of a few meters in length, accelerating particles to hundreds of MeV, seem quite real.
Third, the great advantage of this acceleration technology is that it is completely optical and practically continuous (that is, it works for a long time on a pulsed laser with millions of pulses per second). It is not necessary to suffer with electromagnets, and even more so with superconductors, as in conventional accelerators; Do not shoot with super-powerful light pulses and burn the foil, as in laser; It is not necessary to maintain plasma in special chambers and create oscillating bubbles with a field in it. The correct glass, vacuum and light are all that is required for dielectric acceleration. Moreover, since there are no moving parts in this optical device, there is another advantage - an exceptional speed when switching modes.
Fourthly, the described technique allows not only to accelerate particles, but also to make other manipulations with beams - to turn them and even to focus. As a result, an amazing design seems to be realized, which can be called an "accelerator-on-a-chip" (Figure 5, right). Inside a single glass, all the key elements of the cyclic accelerator will be executed, and the user will only need to inject the particles and shine with the laser beam in the right place at the right time. In the light of all these described achievements, such cheap and ultraportable accelerators no longer seem to be anything fantastic.
Such an interesting combination of advantages makes it possible to think about new installations, which at first did not come to mind. For example, if you do not pursue energy, but ensure compactness and monochromaticity of the electron bunch, as well as controlled on-off and deflection, you can get a new (and very compact!) Tool for ultrafast electron diffraction or time-resolved electron microscopy.
Of course, some of the implementation difficulties are still here. For example, it is required to provide suitable beam parameters at the input to the structure; Still, he needs to squeeze into the gap less than a micron and do not go too far. For the ideal operation of the dielectric accelerator, it is also required that the electron beam at the input be divided into ultrashort and equally spaced clumps. However, since already there are working devices, these difficulties, apparently, are not fundamental.
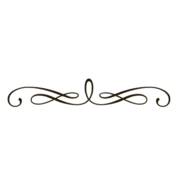
References for Text and Images:
- https://journals.aps.org/prl/abstract/10.1103/PhysRevLett.111.134803
- https://www.sciencedaily.com/releases/2017/02/170202163030.htm
- https://physics.aps.org/articles/v6/106
- https://physics.aps.org/articles/v9/132
- http://www.slac.stanford.edu/cgi-wrap/getdoc/slac-wp-117.pdf
- https://www.etit.tu-darmstadt.de/fachbereich/news_englisch_etit/archive/news_archiv_details_en_107904.en.jsp
- http://www.laserfocusworld.com/articles/print/volume-49/issue-11/world-news/laser-driven-particle-accelerators-on-chip-dla-is-precursor-to-tabletop-particle-accelerator.html
- http://newscenter.lbl.gov/2009/12/22/accelerators-tomorrow-part2/
Support @steemstem and the #steemstem
project - curating and supporting quality STEM
related content on Steemit
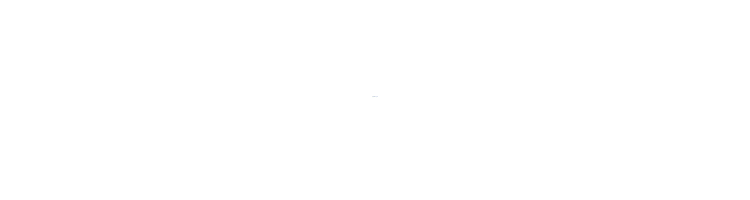
Can it be used to make gold? Do u think it will be economically feasible? thanks in advance.