The Cosmic Distance Ladder - A Quiet Gem Of Science
I first learned about the Cosmic Distance Ladder when I was a kid and found it amazing that while being stuck on the Earth astronomers could figure out the distances to objects right up to the edge of the observable universe.
The Cosmic Distance Ladder is truly one of the quiet gems of science. Using observational data and scientific deduction of several astronomical object types and phenomena, a ladder of overlapping distance regimes can be painstakingly built up.
This post is about that distance ladder and also a review for me to see what new rungs have been added to that ladder over the years since I first learned about it.
Distance Units
Before we start we first need to understand astronomical distance units. These are typically measured using the speed of light as the primary tool. The speed of light is approximately 300,000 kilometres per second in metric or 186,000 miles per second in freedom units.
The distance that light travels in a hour is called a light-hour. The distance that light travels in a day is called a light-day and you guessed it, the distance that light travels in a year is called a light-year.
Almost no one ever talks about the actual number of kilometres (or miles) in a light-year because the numbers will be simply too large and unwieldy to use in any practical sense.
The other distance unit used in astronomy is called the parsec ("second of parallax"). It is related to parallax as the Earth swings from one side of its orbit to the other. More formally, the original definition of a parsec is the distance at which one astronomical unit will subtend an angle of one arc-second. However it was recently re-defined to be exactly 648000/π astronomical units (reference).
In short, it turns out that a parsec is equal to ~3.26 light-years.
Radar Ranging (Good Out To A Few Light-Hours)
The first rung on the ladder is radar ranging. The concept is simple, we know the speed of light so we simply send a radar ping out to an astronomical object such as the Moon, Venus, Mars, a moon of Jupiter or Saturn etc. and measure the time for the echo of that ping to arrive back at the Earth.
The light time to that object is therefore one-half of the measured time and the distance is that time multiplied by the speed of light.
This method is good out to several light hours and it is completely useless for measuring the distance even to the nearest star.
Parallax (Good Out To ~300 Light-Years)
Hold your thumb out at arm's length, look at it and close one eye. Fix that thumb's position against the far distant horizon.
Got that image memorized? Okay good. Now close that eye and open the other one. Your thumb will appear to shift position against the far distant horizon background. This effect is called parallax and it depends on simple trigonometry.
If you know the distance between your pupils you can calculate the distance to your thumb by measuring the angle that your thumb appeared to shift against the un-shifting and distant horizon.
Astronomers can take advantage of this phenomenon of trigonometry as the Earth orbits around the Sun and shifts from one side of it's orbit to the other. In this case the distance between our 'pupils' is the width of the Earth's orbit (i.e. 2 astronomical units or AU)
Astronomers take a photo of a nearby star in, let's say, January and then they take another photo of the same star 6 months later in June. The nearby star will appear to shift position against the backdrop of more distant stars. In actual fact it hasn't, it is only the parallax phenomenon due to the Earth moving its position to the other side of its orbit.
We know the distance from one side of Earth's orbit to the other, the two photos will provide the change in the star's angular position and then using some trigonometry scientists can figure out its distance in units of AU which they will convert to light-years.
This technique is good out to maybe 300 light-years or so, although it is said that the Hubble Telescope can measure parsecs for some bright stars out to 20,000 light-years. In the 2020's the European Space Agency will be launching the Gaia satellite that will be able to do the same but for all moderately bright stars.
Main Sequence Star Brightness Fitting (Good Out To ~10,000 Light-Years)
It turns out that stars are fairly predictable physical systems. The brightness of a star (i.e. one that is not being interfered with by another star) will depend primarily on its mass, composition and its age. This star will evolve as its ages getting brighter or dimmer over the aeons and as it uses up its primary fuel (hydrogen).
These are called the Main Sequence stars and it means that astronomers can calculate with some accuracy what the absolute brightness of a star should be.
We also know that the brightness of an object varies inversely with the square of its distance from us. Combine these two pieces of knowledge and the distance of many stars can be calculated if we measure it's apparent brightness in the sky and if we can get a good calculation of its real (i.e. absolute) brightness.
This method for estimating distances is claimed to be good out to about 10,000 light-years.
Novas (Good Out To ~3,000,000 Light-Years)
Some types of nova (exploding stars) are also fairly predictable physical systems and can therefore be used as fairly useful distance indicators. Astronomers have found that the brightness of nova is bimodal (i.e. they tend to fall into one of two peaks).
The most common peak is at an absolute magnitude of -8.8 and the less common peak is at an absolute magnitude of -7.5. They are also found to have approximately the same absolute magnitude about 15 days after their peak brightness (−5.5).
This means that if you measure the apparent magnitude of a nova and compare it against it absolute magnitude you can get a good estimate of its distance using our good friend the inverse square law for brightness.
This technique is said to be good out to about 3 million light-years.
Cepheid Variables (Good Out To ~10 Million Light-Years)
Remember what I said about regular main sequence stars and nova being fairly predictable physical systems? Well the same thing is true for certain types of variable stars. In particular the Cepheid variable stars named after the prototypical star Delta Cephei in the Cepheus constellation.
This class of variable stars are very bright which happens to be quite useful if you want to see them at very large distances. These stars pulsate radially, this means that as they get larger they will also get dimmer and as they get smaller they will get brighter. The changes in their brightness have nice stable periods and amplitudes, in fact it turns out that there is a very strong relationship between a Cepheid star's brightness and it's pulsation period.
This makes this class of star a good standard candle for measuring distances at very large distances, even in other galaxies. The first Cepheids were measured by Henrietta Swan Leavitt in the Magellanic Clouds (satellite galaxies of our Milky Way).
The Cepheid distance measurement technique is claimed to be useful out to about 10 million light-years.
White Dwarf Supernovae (Good Out To 10 Billion Light-Years)
Novae are exploding stars and are incredibly energetic however there is a class of exploding star called supernovae whose power dwarves even these cataclysms. One type of supernova is called the White Dwarf Supernova or Type Ia Supernova.
This supernova type can occur in a binary star system if one of the stars is a white dwarf and the other star has evolved to a point in its life where it swells up and spills its gas onto the surface of the white dwarf. Eventually the white dwarf will reach a critical mass and explode.
The mechanism and star evolution is more involved than this simple description and can be found here if you are interested. The important point is that these supernovae have predictable light curves and can therefore be used as standard candles.
We already know from above that if you can use something as a standard candle you can peg down its absolute brightness. Then if you measure its apparent brightness in the sky, apply the inverse square brightness law you can get a good estimate of that object's distance.
The Type Ia Supernova distance measurement technique is claimed to be useful out to about 10 billion light-years. These supernovae have been observed and measured in many galaxies and have therefore been very useful in determining the distances to their host galaxies.
Tully-Fisher Relation (Good Out To 10 Billion Light-Years)
The Tully-Fisher Relation is a measured correlation that has been found between the mass of a spiral galaxy (which can be estimated by its luminosity ) and the angular velocity of that galaxy. In this technique an entire galaxy is being used as a standard candle which is quite an interesting concept.
The Tully-Fisher distance measurement technique is claimed to be useful out to about 10 billion light-years. However looking at the scatter in the data (see image above) and the vagueness of the fit of the correlation curve it really does not seem to me to be the best distance measurement technique out there.
Hubble's Law Of Expanding Space-Time (Good For Distances Beyond 300 Million Light-Years)
My favourite astronomical distance measurement technique has to be the Hubble Law. It relates the expansion of the space-time fabric of the Universe to distance from our solar system and is the most "science-fictiony" of all the techniques discussed so far.
This relation is based on the observation that distant galaxies are found to be moving away from us as measured by the redshift of the light that they emit. The farther the galaxy is (as determined by Type Ia supernova) the greater the redshift and thus the faster it is moving away from us. The effect was discovered by Edwin Hubble in 1922 and has been used to measure the distances of objects right out to the edge of the observable universe.
The technique is less useful for closer galaxies because the expansion of space-time is smaller the closer you are to something (space-time expansion is a cumulative effect). This means that the unique velocity (peculiar velocity) of a nearby galaxy can dominate and overwhelm the Hubble relationship. Only at very large distances will the expansion of space-time be much larger than the velocity of any given galaxy.
Closing Words
There are a few other distance measurement techniques that I did not mention but the most important and most reliable ones have been discussed in this post.
I find it truly remarkable that one can go from trigonometry, to variable stars, to supernovae and then finally to the expansion of the Universe itself to build up an interleaving system of measurement techniques that can get us all the way to the edge of the observable Universe.
This is why I said at the beginning of this post that the Cosmic Distance Ladder is truly one of the quiet gems in all of science.
Thank you for reading my post.
Post Sources
[1] The Cosmic Distance Ladder.
[2] The Speed of light.
[3] The Parsec.
[4] The Astronomical Unit.
[5] Gaia Space Mission.
[6] Main Sequence Star Diagram.
[7] Novae as distance indicators.
[8] Cepheid variable stars.
[9] Type Ia Supernova.
[10] Tully-Fisher Relation.
[11] Hubble Law of Space-Time Expansion.
[12] Cosmic Ladder Reference Image 1.
[13] Cosmic Ladder Reference Image 2.
[14] Cosmic Ladder Reference Image 3.
[15] Cosmic Ladder Reference Image 4.
.
No but I just did some digging and it looks very interesting. Thx for the tip.
Maybe I will need to revise this post of mine I did 3 months ago:
Are The Constants Of Physics Actually Constant?
Congratulations! Your post has been selected as a daily Steemit truffle! It is listed on rank 5 of all contributions awarded today. You can find the TOP DAILY TRUFFLE PICKS HERE.
I upvoted your contribution because to my mind your post is at least 36 SBD worth and should receive 134 votes. It's now up to the lovely Steemit community to make this come true.
I am
TrufflePig
, an Artificial Intelligence Bot that helps minnows and content curators using Machine Learning. If you are curious how I select content, you can find an explanation here!Have a nice day and sincerely yours,
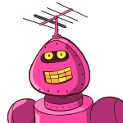
TrufflePig
Thx pigbot you are a good bot. Have an upvote to help support your work.