Cytoplasmic Determinants
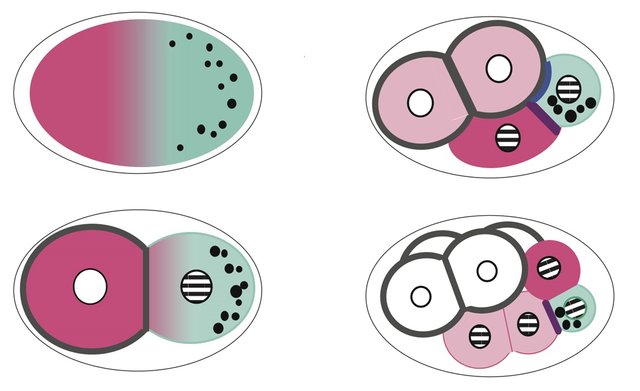
Developmental processes not only create many cells but also cause those cells to differentiate into many different types of cells.
A zygote can give rise to any type of cell, but its daughter cells eventually cannot. Several theories have been advanced to explain why, how, and when the fate of a cell becomes more restricted, with that cell only able to develop into a specific type. Experiments designed to test these theories eventually led to the recent development of cloning and point to the great promise of stem cell research. We will outline the two gene regulation mechanisms that control differentiation and discussing the first, cytoplasmic segregation.
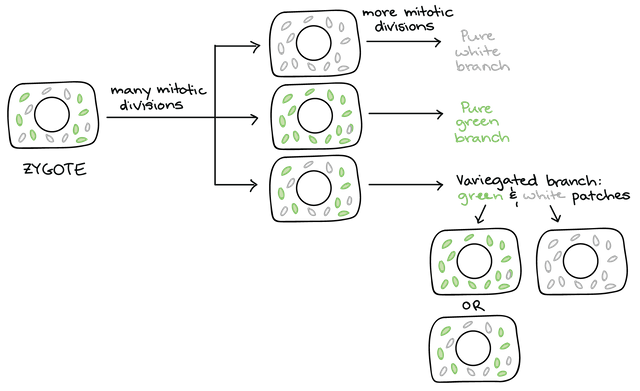
In addition to creating many cells from one, developmental processes must yield many different types of cells.
A zygote is said to be totipotent, meaning that the cell has the potential to give rise to the entire organism, including all of its different cell types and structures. As development proceeds, however, the potential of individual cells to specify an entire organism generally diminishes. In some organisms, snails for example, the two blastomeres that result from the very first cleavage division already have lost the potential to develop into the whole organism on their own. In other organisms, the blastomeres resulting from the first few cleavages can be separated, and each will then develop into an entire normal organism. In mammals, for instance, each of the cells in the eight-cell stage (after three cleavage divisions) can develop independently into a complete adult. At some point in the development of all organisms, however, the potency of the blastomeres arising from cleavage begins to be reduced. After some number of cell divisions, individual blastomeres can no longer develop into complete adults.
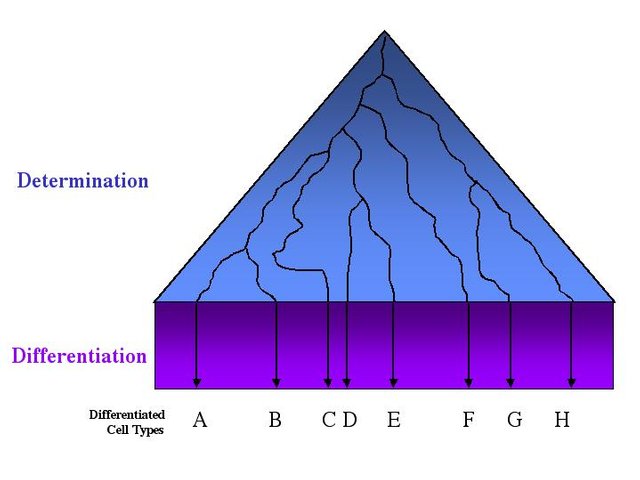
As a cell’s potency is reduced, its fate becomes more restricted.
This restriction of cell potential is called determination. In developmental biology, determination means commitment—cells become committed to specialize in a particular way. A cell may be determined long before it shows any specialization in its biochemical, physiological, or morphological properties. Determination is not a sudden occurrence in the life of a cell, however; it is a stepwise process. Even after blastomeres have lost their potential to develop into a whole organism, they may still be able to develop into several different kinds of cells. Although their potential has become restricted, the fate of these blastomeres is not entirely determined. At some later stage, however, the progeny of these cells will have an even more restricted set of possible outcomes. Determination represents a progressive restriction of a cell’s developmental potential. When a cell finally achieves its developmental endpoint, it is said to have differentiated. Most of the cells in an adult organism are highly differentiated, with distinct physical, biochemical, and physiological properties that enable them to perform specific functions.
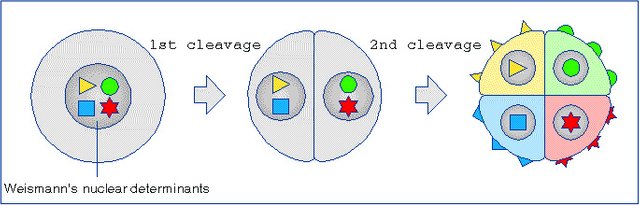
Several theories have attempted to explain cell determination and differentiation.
We know today that all cells in an organism have the same genetic material and that determination and differentiation must have something to do with how those genes are turned on and off. Before biologists knew about genetic mechanisms, however, it seemed equally possible that determination and differentiation happened as a result of cells receiving a progressively limited set of genes over the course of development. August Weismann, in his germ plasm theory, assumed that there must be some sort of “determinants” that were passed on as cells divided. Weismann suggested that a fertilized egg contained all the determinants needed to direct the development of an entire organism. He called this complete set of instructions the germ plasm. Weismann proposed that as cells divide during cleavage, they receive different subsets of the germ plasm.
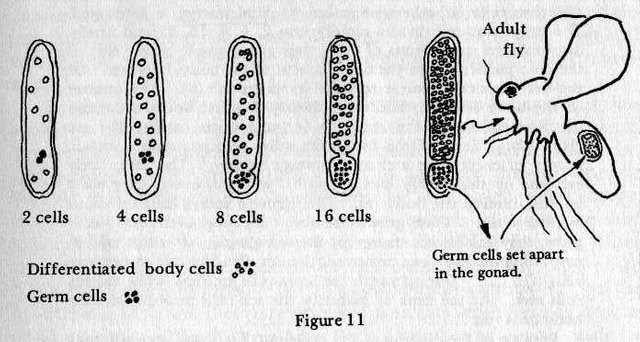
However, this theory presented a difficulty:
If the cells of an adult organism were created by dividing up the entire germ plasm, how could that adult reproduce itself? Weismann suggested that, at the very beginning of development, a small group of germ line cells, each with an entire copy of the germ plasm, is set aside for reproduction. A few years after Weismann published his theory in 1883, Wilhelm Roux fertilized frog eggs in the lab and allowed them to go through the first division of cleavage. He then killed one of the resulting two blastomeres. Roux observed that the remaining cell was unable to develop into a complete frog, which seemed to support Weismann’s germ plasm theory. There was a problem with this experiment, however; although Roux killed one cell, the dead cell stayed in contact with the living cell. We know now that proteins on cell surfaces act as signals during development, so the presence of the dead cell may have affected the development of the remaining living cell.
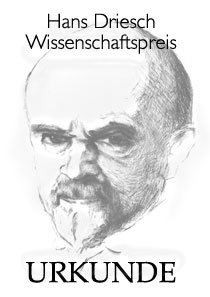
In the early 1890s, Hans Driesch performed a similar experiment but physically separated the two cells.
When separated, the two blastomeres would each develop into complete adults. Many biologists in the 1890s and early 1900s performed similar experiments, with the general result that blastomeres often retained totipotency for at least the first few divisions of cleavage. Though these experiments ruled out the germ plasm theory in the strictest sense, they did show that blastomeres eventually lost totipotency. Only in the 1950s did it start to become possible to test whether differentiated cells all retain the same genetic material, by transplanting the nucleus of a differentiated cell into an egg cell. However, the crudity of the technique at first made it difficult to find a definitive answer.
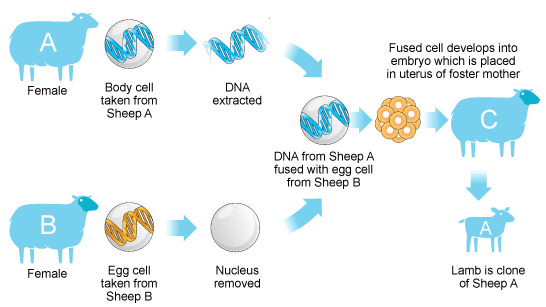
In 1997, Ian Wilmut and colleagues successfully transplanted a nucleus from a six-year-old female sheep into an enucleated egg taken from another female.
The egg with the transplanted nucleus was then implanted into the uterus of a third female. The result was the birth of the world’s most famous lamb, Dolly. Dolly grew up normally and was even able to produce offspring of her own, demonstrating conclusively that the nuclei of even the most differentiated cells in an adult organism retain an entire set of genetic material and finally putting the germ plasm theory to rest.
- Of course, the reason many people have heard of Dolly is because she was the first example of a mammal being cloned, through the process of nuclear transplantation.
- Clones are, by definition, genetically identical individuals.
- Since Dolly was cloned in 1997, many other mammals, such as mice, cats, cows, and other farm animals, have been cloned successfully. In theory, there is no reason why humans could not eventually be added to this list.
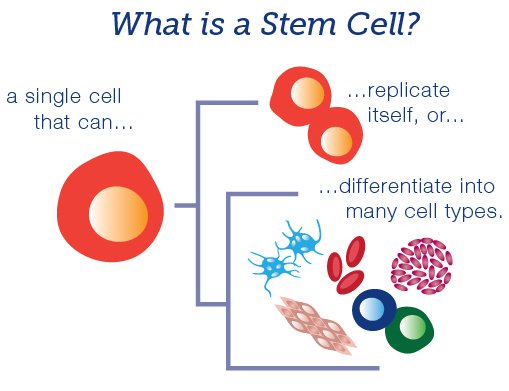
Determination and the developmental potential of cells also lead into another much-discussed topic stem cell research.
A stem cell is any cell that remains relatively undifferentiated in an organism. Though most cells in an adult organism are highly differentiated, some tissues need frequent replacement or repair and retain cells that can still divide and differentiate into different cell types. One reason stem cell research is so interesting is its potential application to medicine.
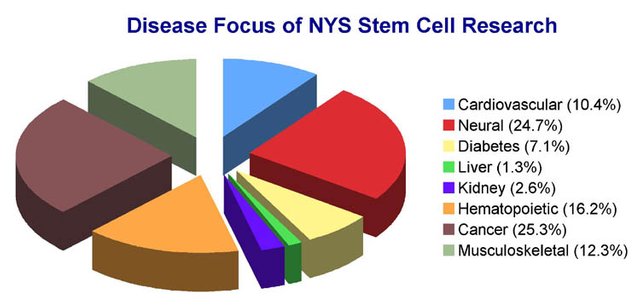
The long-term goal of this research is to use stem cells to replace damaged cells in such systems as the brain, where cells are not normally replaced. Though adult stem cells are relatively undifferentiated, they generally retain the ability to differentiate into only a few types of cells. The stem cells that have the most potential to differentiate are found, instead, in very young embryos. Embryonic stem cells can be very close to retaining totipotency. The difficulty is that embryonic stem cells must be derived from human embryos, specifically from surplus embryos donated by infertile couples. This potential use of human embryos involves serious ethical and political considerations that are still under debate.
Because the genomes of differentiated cells remain the same, differences between cell types must be the result of gene regulation mechanisms. We have seen that the presence or absence of transcription factors is ultimately what controls gene expression; therefore, we must ask what causes differences in the distribution of transcription factors in different cells in the developing organism. The distribution of transcription factors in a cell’s cytoplasm is called the cytoplasmic environment.
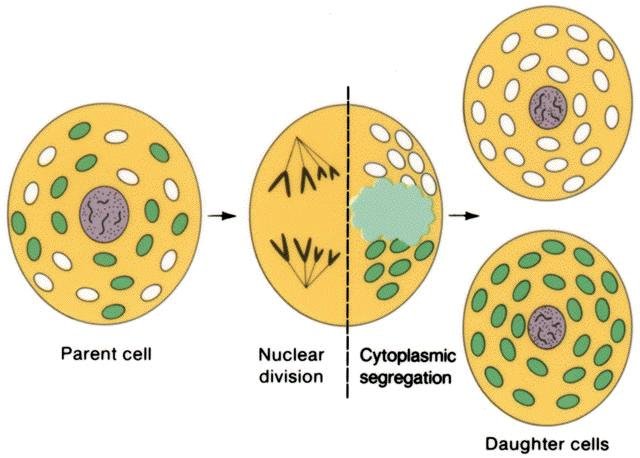
Two main mechanisms affect the cytoplasmic environment during development.
The first of these mechanisms is called cytoplasmic segregation. Because of the asymmetry of the zygote, maternal determinants may be divided differently among blastomeres during early cleavage, depending on the orientation of cell divisions. These determinants are largely responsible for controlling the earliest phases of development, and cells with different complements of determinants will follow different developmental trajectories.
The second mechanism is called induction, which refers to the influence of neighboring cells on the development of an embryonic cell. Induction becomes especially important in later stages of development, as the structure of the developing organism becomes increasingly complex.
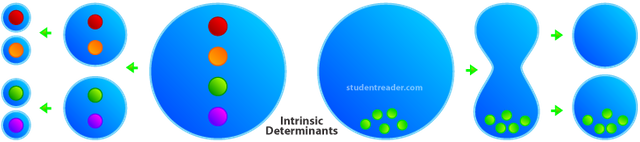
In many organisms, cytoplasmic determinants, not the expression of the organism’s own genome, play the largest role in the very earliest stages of development.
The mRNAs and proteins already stored in the egg by the mother are responsible for controlling cleavage and the formation of the blastula. Blocking transcription factors in a developing organism will generally make no difference until the gastrulation phase.
The asymmetric segregation of maternal determinants during cleavage is responsible for determining the fundamental organization of the developing embryo. Classic experiments done on sea urchins in the late 1920s by the Swedish biologist Sven Hörstadius illustrate this point.
Sea urchin blastomeres (or even zygotes) divided along the longitudinal axis will develop normally; those divided along the equatorial axis will develop into misshapen larvae or not develop at all. This is because a longitudinal division preserves the original distribution of cytoplasmic determinants, whereas an equatorial division does not.
Sources: 1
Image Sources:
https://ka-perseus-images.s3.amazonaws.com/267bbbde05f5f120faa095c17f3a20cb1d3a74f4.png
http://www.wormbook.org/chapters/www_asymcelldiv.2/acd2fig5.jpg
http://biology.kenyon.edu/courses/biol114/Chap11/Slide1.jpg
http://www.mun.ca/biology/desmid/brian/BIOL3530/DB_01/fig1_7.jpg
http://custance.org/Library/SOTW/Part_II/fig11.jpg
http://hans-driesch-preis.org/online/wp-content/uploads/2012/12/urkunde.jpg
http://www.bbc.co.uk/staticarchive/9c7cc8f9772c8e1087f3da7b1affa4c00bb5109c.gif
https://www.caricord.com/images/image.jpg
http://stemcell.ny.gov/sites/default/files/images/figure2pie.jpg
http://www.cbs.dtu.dk/courses/genomics_course/roanoke/fig22_04.jpg
http://studentreader.com/files/figures/intrinsic-determinants-egg-cytoplasm.png
Bird [Giphy](https://giphy.com/gifs/blue-5lDik5jPpRw2Y)
Thanks #steemit for Donating
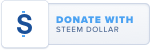
BTC: 1JQWNxziArfaCtxsP3tUQ4VSTBY9jresmt
Zcash: t1fKGLRuwDM4KUTfE9f8nso3dkB9Ew1KUod
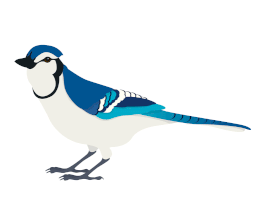
bright congratulations post thanks for sharing
Thanks for reading @jlufer, more great stuff to come.
Thank you for being a part of this platform! #namaste
This is simply a miracle!