Deciphering a hadronic collision such as occurring at the Large Hadron Collider at CERN
As some of you already know it, I have a great interest for the Large Hadron Collider at CERN, or the LHC in short. This interest is of course connected to my work.
The LHC is the largest accelerator complex in the world and the largest particle physics exploration machine made by human beings. By exploration, I mean that this machine, with its energy and the number of collisions occurring every second, allows us to probe phenomena that are potential signs of physics beyond the Standard Model, or new physics.
In this post, I would like to detail what is going on during a typical LHC collision. It is based on lectures I have given several times at CERN during the last few years. All the figures are taken from a movie that has been designed for educational purposes.
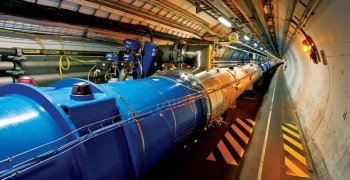
[image credits: CERN]
COLLIDING PROTONS
While a proton can be seen as a sphere with two up quarks and one down quark inside, this simplistic picture turns out to be incorrect when the proton is accelerated as at the LHC.
It must be considered more like a dynamical pancake made of quarks, antiquarks and gluons that are created and annihilated all around the place by virtue of the strong interactions (yum yum). This picture stems from quantum chromodynamics (that is the theory of the strong interactions).
Two colliding protons are thus equivalent to two colliding pancakes, as illustrated in the figure below. The grey bubbles are the three valence quarks that are those we refer two when one says that the proton is made of three quarks. All other bubbles are those quarks, antiquarks and gluons popping up from everywhere I just mentioned.
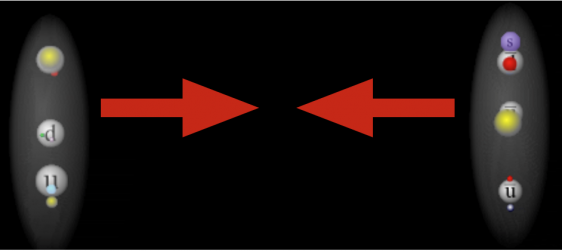
[image credits: from this educational movie]
During a collision, what is happening most of the time is … nothing.
The two protons cross each other and continue their way unnoticed. Like in the picture below where the constituants of both protons just pass through each other like ghosts.
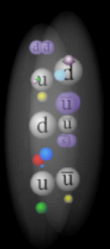
[image credits: from this educational movie]
THE HARD SCATTERING PROCESS
Once in a while, one of the constituants of one of the protons will interact with one of the constituants of the second proton. And this starts to be interesting…
Massive particles can be produced since our colliding protons are very energetic. Special relativity indeed shows the rest: a lot of energy means that very massive particles can be created. This is what is called a hard scattering process.
In the example below, a pair of top quark and top antiquark (or antitop) is created. Yeah, I like top quarks. One can also admire once again the dynamical form of the protons: the quark, antiquark and gluon content of the proton is not static.
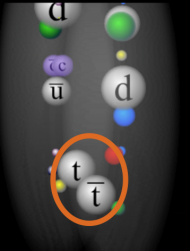
[image credits: from this educational movie]
MASSIVE PARTICLE DECAYS
Heavier particles are however unstable. In the case of our top quarks, weak interactions imply that they will quickly decay, before they have the time to do anything else.
Both the top and antitop quarks will decay to a W-boson (one of the carriers of the weak interactions) and a bottom quark, as illustrated below.
On the left figure, the antitop has not decayed yet whilst on the second figure, both our top quark and antiquark have decayed.
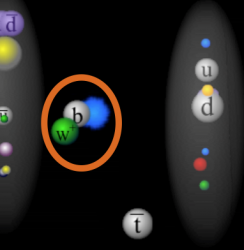
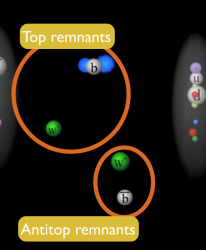
[image credits: from this educational movie]
This decay takes no time at all. Just to give an order of magnitude, the top quark lifetime is of about 1e-25 second, or 0.000…01 second with 24 zeros.
The story of course does not end there, as W-bosons are also unstable particles that will decay right away as well.
Contrary to top quarks which decay almost every time into a b-quark and a W-boson boson, the possible decay modes of the W-boson are more varied.
Let’s forget about antiparticles so that I will make use of the word quark equivalently for a quark and an antiquark. A W-boson can then decay either into a pair of quarks or into a charged lepton (electron, muon, tau) and the associated neutrino.
All these modes are not equally probable, and the five winner W-boson decay modes are up-down and strange-charm decays (with a rate of about 30% each) and the electron-neutrino, muon-neutrino and tau-neutrino ones (with a rate of about 10% each).
In the figure below (let's ignore the red arrow for the moment), one W-boson (the one issued from the top quark) has decayed into a pair of charm and strange quarks, and the other one (originating from the top antiquark) into an electron and an invisible neutrino.
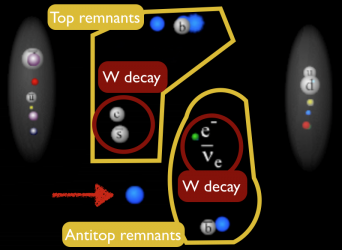
[image credits: from this educational movie]
PARTON SHOWERING
On the last figure, one can also notice a single blue dot (with a red arrow pointing to it) that does not seem to belong to any decay chain. This is not a mistake and this guy is really there.
Together with the other blue spots that are included within the two decay chains, we have four of these guys on the figure. And some of these guys were already there on the previous figures if you check carefully.
What we are witnessing here is the phenomenon of parton showering. Imagine you take a shower, but of quarks and gluons (that are both generically called partons) … but no… Although I am sure that after the reading of this paragraph, it will be easy to guess where the name ‘parton showering’ comes from.
More seriously, these blue dots result from quantum chromodynamics (the theory of the strong interactions).
Very energetic quarks and gluons are strongly interacting particles, and they can thus radiate other quarks and gluons, lowering in this way their energy.
The energy decrease goes on until it reaches a lower threshold where another type of phenomenon starts: the hadronization process. At that moment, the results of our collision may look like the picture below, with a bunch of extra partons that have appeared.
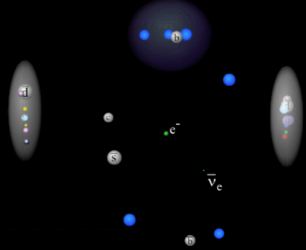
[image credits: from this educational movie]
HADRONIZATION
There is no free quarks and gluons observable in nature. As a consequence, we can only observe composite states, called mesons and hadrons, that are made of quarks and gluons.
The associated formation mechanism is called hadronization and stems from a property of the strong interactions called confinement. The name comes from the fact that the quarks and the gluons are confined into hadrons and mesons.
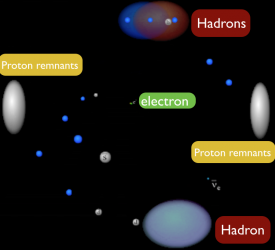
[image credits: from this educational movie]
On the above figure, one can notice that three new hadrons have been formed, which gives 5 hadronic states in total with the proton remnants. The electron is still there (notice the scale that is much larger than before) and we still have a bunch of quarks and gluons that have not hadronized yet and thus continue radiating (new blue and grey dots in the figure).
Many hadrons are moreover unstable and will thus decay into other hadronic systems. This also holds for the proton remnants that will eventually decay too.
JET FORMATION
The final picture gets more complicated, and two of the final steps of the collision history are shown below.
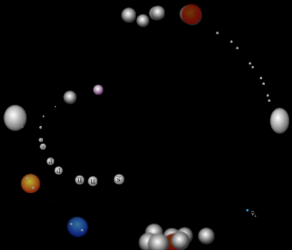
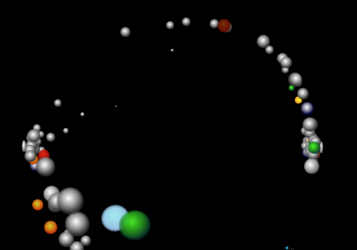
[image credits: from this educational movie]
The protons remnants are still intact on the left, and decayed on the right. Extra parton showering activity is shown oin the left picture, which gives rise to more quarks that again hadronize and decay (see the right figure).
All final state hadrons are actually clustered into disjoint groups. Each group is what we call a jet of strongly interacting particles, or simply a jet.
It is also interesting to recall that we have an electron somewhere, but at a scale invisible on such a figure. The accompanying neutrino is also there, but it will leave no track in the detector.
SUMMARY
Although an LHC collision is a complicated process, we are understanding all steps pretty well.
One can consequently design related computer codes so that it is now very easy to simulate, even on a simple laptop, any hard process in the context of any particle physics model, as well as the parton showering and the hadronization processes.
At the end of the day, the collision final states that are searched for are made of jets, electrons, muons, tau leptons, photons and missing energy. Those are the only objects we can play with to reconstruct what has happened…
This is a great description :-) Makes me laugh a little. Did you explain like this during your lecture?
For CERN summer students yes. For master students definitely not as 'ghost' has a special meaning in quantum field theory. It is good not to even try confusing the students :D