What is the Klein-Kaluza theory and it's implications
The Klein-Kaluza space
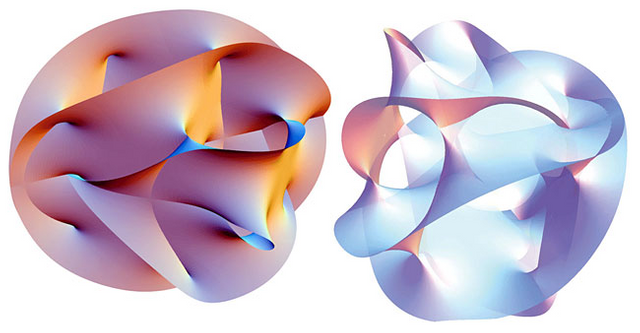
source
The day before his death, Einstein asked to be given his latest calculations on the combined field theory. For thirty years he tried fruitlessly to change the general theory of relativity so that it covered electromagnetic forces as well. One of the most promising options arose in Einstein in 1919, at the very beginning of his search, while he parsed the mail. The idea visited his mind not directly, but through the letter of a pauper mathematician named Theodore Kaluza.
In the letter, Einstein found a proposal on how to combine electromagnetic forces with gravitational forces. This theory had one little strangeness. Einstein wrote in response: "The idea of creating [a unified theory] by means of a five-dimensional cylindrical world never occurred to me ..." Five-dimensional cylinder? But to whom could this ever come to mind? Nobody knows how Kaluza thought of this before, but Einstein added in the same letter: "Your thought is extremely sympathetic." Now we understand that Kaluza has overtaken time, but he was greedy for the measurements.
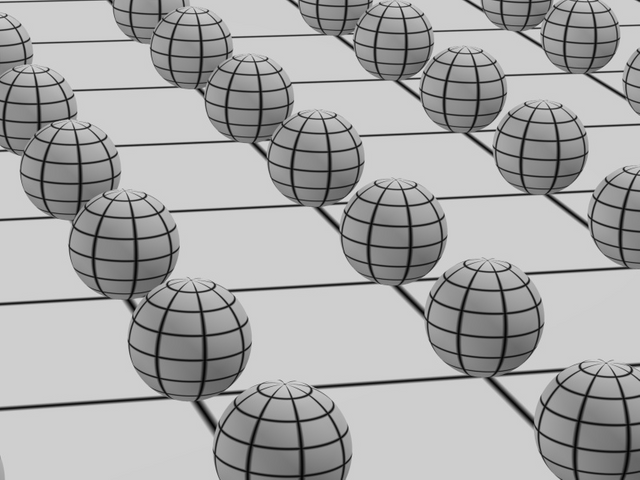
source
The general theory of relativity described how matter affects space through a metric whose components - g-factors - tell how to measure the distance between neighboring points based on the difference in their coordinates. The number of g-factors depends on the number of space measurements. For example, there are six of them in a three-dimensional space. In the plane, the distance equals (the difference between the coordinates x) 2 + (the difference between the coordinates y) 2 + (the difference between the coordinates z) 2, ie gxx, gyy and gzz are all equal to 1, and the factors corresponding to the cross ones are gxy, gyz and gxz - all are equal to zero and they are not in the equation. In a four-dimensional non-Euclidean space, ten independent g-factors emerge from the general theory of relativity (taking into account equalities of the type gxy = gyx), all of which are described by Einstein's equations. Kaluza first realized this: if you take five measurements, there will also be g-factors that correspond to the additional measurement.
Further, Kaluza asked himself the question: if one extensively extends the Einstein field to five dimensions, what equations are obtained for additional g-factors? The answer is stunning: Maxwell's equations for the electromagnetic field come out! Starting with the fifth dimension, electromagnetism suddenly appears in the theory of gravity. Einstein wrote: "The formal unity of your theory is striking."
Of course, the interpretation of the metric of the complementary measurement as a physical electromagnetic field requires some fuss with theory. And what, by the way, is there with that little strangeness - an additional dimension? Калуца asserted, that it certainly on length, and it is even more exact - such small that we and did not notice it even if would swarm inside. Moreover, Kaluza said that the new dimension has a new topology: in it, instead of a straight line, there is a circle, that is, it closes on itself, is folded (and therefore, unlike a finite line, does not have ends). Imagine Fifth Avenue with zero width - in the form of a simple line. In the new dimension of Kaluza, the intersecting streets will turn into circles that cut from Fifth Avenue. Of course, intersecting streets occur at intervals of a quarter, but an additional dimension is at every point along the avenue. Thus, if you add a new dimension to a line, it will not grow in circles, but will turn into a cylinder like a garden hose. Only very thin.
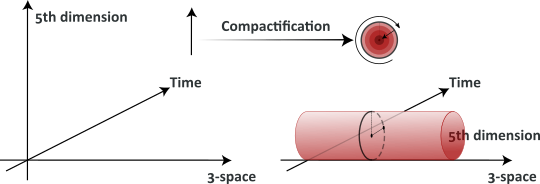
source
In fact, Kaluza argued that gravity and electromagnetism are in fact components of the same thing, but they look different because we observe some averaged impalpable motion of a tiny fourth dimensional dimension. Einstein doubted the theory of Kaluza, but after a while he changed his mind and in 1921 helped Kalutse publish his theory.
In 1926, Oscar Klein, assistant professor at the University of Michigan, regardless of Kaluza proposed the same theory, but with some improvements. One of them is the realization that this theory leads to the correct equations of particle motion, if in this mysterious fifth dimension the particle has definite momentum values. These "allowed" values were multiples of a certain minimum momentum. Assuming, as Kaluza did, that the fifth dimension is self-contained, one can apply the quantum theory in order to calculate from the minimum momentum the possible value of the "length" of this folded fifth dimension. If it suddenly became clear that the measurement is of a foreseeable, macroscopic size, the theory would be in jeopardy, since we do not observe this dimension at all. But the size was 10-30 centimeters. No problem. Measurement is hidden from the eyes be healthy.
The Klein-Kaluza theory hinted at a formal connection between theories, but not on a structure that immediately provided something completely new. For the next few years, physicists were looking for other predictions that this theory could give, in about the same way that Klein was discussing the dimensions of the new dimension. They managed to find new arguments, which seemed to imply that with its help it is possible to predict the ratio of the mass of the electron and its charge. However, the result of the prediction strongly disagreed with reality. Somewhere halfway between this difficulty and the strange prediction of the fifth dimension, physicists have cooled to a new theory. Einstein returned to her in 1938 for the last time.
Kaluza, who died a year before Einstein, almost did not advance further. But something from his fledgling theory to him in a big way. When he wrote to Einstein, he was 34 and he had already kept his family for ten years on the salary of the privat-docent (an approximate analog of the assistant professor) in Koenigsberg. This salary is best described in terms of mathematics dear to his heart: for each semester he received 5 times x times from German marks (or, strictly speaking, gold marks), where x was equal to the number of students in his class, and y - to the number lecture hours weekly. As a result, it turned out about 100 marks a year. In 1926, Einstein called such conditions of life "schwierig", which roughly means "only dogs can live like this." With the help of Einstein Kaluz in 1929, he finally received a professorship at the University of Kiel. He moved to Goettingen in 1935, where he became a full professor. There he lived another nineteen years assigned to him. However, until the 1970s, no one seriously considered the possibility of new measurements.
The birth of strings
Who knows when the inspiration will flow? It is still impossible to know where it will lead. The history of the string theory begins at the top of a 750-foot mountain in the Mediterranean. The city is called Erice, that in Sicily - unhurried, hot, its streets are narrow and dressed in an ancient stone. Erice was Erice, when Thales still roamed the Earth. Nowadays the city is famous primarily for its "Centro Ettore Majorana" - a cultural and scientific center, where summer schools for about a week span are held for several decades. The schools of Ettore Maggiore are assemblies of senior students and junior faculty members, where they meet with leading scientists of different fields and listen to lectures on the most advanced topics of science.
In the summer of 1967 one of such advanced topics was the approach to the theory of elementary particles called "S-matrix theory." Gabriele Veneziano, an Italian graduate of the Weizmann Institute in Israel, was in the audience and listened to his intellectual hero, Murry Gell-Mann. Gell-Mann will soon receive for his discovery of the quarks the Nobel Prize - at that time they were considered as internal components of a family of elementary particles called hadrons (the same family includes a proton and a neutron). The inspiration that Veneziano acquired in that lecture will inspire him in a few years to create the beginning of the string theory. The theme of the then Gell-Mann lecture was the patterns of the mathematical structure of the S-matrix.
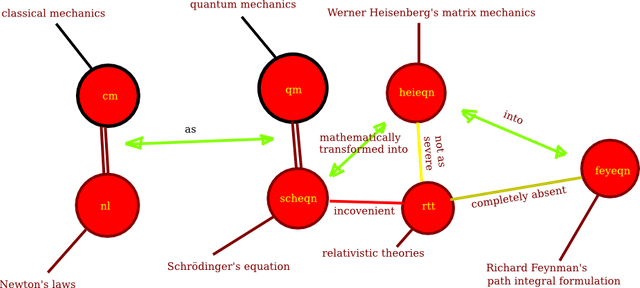
source
The S-matrix was invented by Heisenberg, for the first time in 1937 John Wheeler applied, and its flowering occurred in the 1960s, and was provided by his physicist from Berkeley Geoffrey Chu. The letter S is designated as "scattering", since the main way of studying elementary particles by physicists is as follows: physicists accelerate particles to frenzied speeds and energies, after which they vpapyvayut them each other and see exactly which bells fly in all directions. Just about how to study the device of a car by organizing car accidents.
In small accidents, it is possible to tear off something boring, like a bumper, but at the racing speed, the eyes of a close observer will be presented with the flight of even the bolts and nuts that are most tightly screwed into the passenger seat. But there is one big difference. In experimental physics, having screwed the "Chevy" into "Ford" with the swing, you can get the components from the "Jaguar" on the output. Unlike cars, elementary particles can turn into each other.
When Wheeler developed the scattering matrix, a considerable body of experimental data had already gathered-and continued to accumulate-but a successful quantum theory of creation and disappearance of elementary particles did not exist even in the part of electrodynamics. The S-matrix was a black box into which one could put something-the definition of colliding particles, their pulses, etc.-and obtain similar data at the output, but for the newly emerged particles.
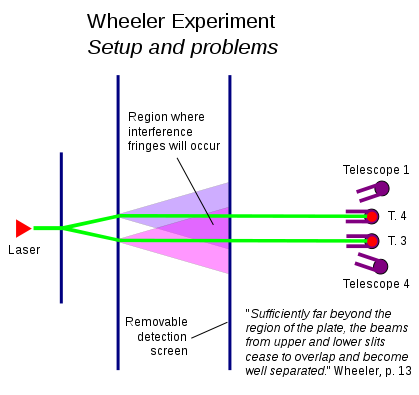
source
In general, the theory of particle interaction was required to construct the scattering matrix, that is, the interiors of the black box. But even without theory, something can be said about the S-matrix - based only on natural symmetries and general principles such as consistency with the theory of relativity. The salt of the S-matrix approach was to find out how far you can go on some of these principles.
In the 50's and 60's of the last century, this approach was almost a craze. In his lecture in Erich, Gell-Mann described some striking regularities, called dualities, which can be observed in the collision of hadrons. Venetiano wondered if such patterns would arise in a more general case. After a year and a half, he realized: all the mathematical properties of the scattering matrix that he considered are inherent in one simple mathematical function, the Euler beta function.
The theory of Veneziano (the dual model of Veneziano) was a startling discovery. Why would a potentially complex scattering matrix take on such a simple elegant form? But this turned out to be the first mathematical miracle in the series of many that would later appear regularly in the string theory - just such beautiful results convinced Schwartz that he was not wasting his life on string theory.
The result obtained by Venetiano seemed to physicists so elegant that it inspired them to a completely non-S-matrix question: how are the particle collision processes organized, because of which the scattering matrix is obtained? What about the black box inside? If we could figure this out, the internal structure of the colliding hadrons would clear up, as well as the interaction called the strong one that controls them.
In 1970, Yoichiro Nambu from the University of Chicago, Holger Nielsen of the Niels Bohr Institute and Leonard Susskind of the Yeshiva University, answered the question: it is necessary to model elementary particles not as points, but as tiny vibrating strings.
We discover or invent a theory? Physicists are the children wandering in the twilight in a park with flashlights in search of truth, or are the children with cubes erecting the towers until they fall asleep? Or, in fact, both? Then what kind of duality is it like that of which Gell-Mann spoke, or like the one that waves and particles have?
There are less pleasant synonyms for the verbs "to invent" and "to open". For example, "cook" or "stumble upon". The original string theory - called the bosonic string theory - was uniquely "concocted". It lacked naturalness, it was filled with incredible properties, and it was clearly collected in a heap, only to reproduce the insight that visited Veneziano. But Nambu and his colleagues came across something. They discovered the string theory practically in the same sense that Planck once was a quantum theory. Both came up against the idea: the energy levels can be represented quantitatively, and the particles can be represented as strings; in both cases neither the true meaning nor the breadth of the scope of these ideas were understood, and the formation of a meaningful theory took years. Both came across something that could be a new law of nature - or simply a mathematical grimace. And only years of effort could determine what is what. In the case of quantum theory, it took 25 years - from Planck to Heisenberg and Schrödinger. String theory has already slipped this line.
Support @steemstem and the #steemstem
project - curating and supporting quality STEM
related content on Steemit

Good post bro so please chak my post